Prognostic implications of left ventricular geometry in coronary artery bypass grafting patients
Introduction
As a significant cause of death and disability, coronary heart disease (CHD) can result in myocardial infarction (MI), ischemic cardiomyopathy, and ischemic mitral insufficiency, leading to left ventricular (LV) remodeling (1). Major cardiac remodeling can cause progressive deterioration of cardiac function (2-5). The remodeling process often involves increases in myocardial mass. Irrespective of etiology, LV hypertrophy, and increased LV mass are independent risk factors for cardiovascular mortality and morbidity (6-8). In patients with CHD, MI, or hypertension, LV hypertrophy leads to a 2- to 4-fold increase in the incidence of death or nonfatal complications (6).
Coronary artery bypass grafting (CABG) is an effective procedure for treating CHD. Along with the aging of the population and improvements in percutaneous coronary intervention (PCI), the complexity of patients referred to CABG is increasing, and so too are the rate of the patients with LV remodeling and LV hypertrophy. The current systems for evaluating the outcomes of cardiac surgery, including the European System for Cardiac Operative Risk Evaluation (EuroSCORE) and the EuroSCORE II, do not consider the impact of LV mass and geometry on surgical outcomes, which hold significant prognostic value (9).
Despite the association between the prognosis of CHD, MI, or hypertension and LV hypertrophy, there is still uncertainty about whether LV geometry, left ventricular mass index (LVMi), and relative wall thickness (RWT) have independent prognostic value on the outcomes of CABG (10). This study aims to explore the prognostic value of LV geometric patterns in CABG patients.
Methods
Study design
The institutional ethics board approved the study of Rui Jin Hospital (No. 2018-15), and informed consent was obtained from all patients. We enrolled patients between January 2012 and September 2016. Patients who underwent CABG and had available preoperative echocardiographic recordings met the criteria for inclusion in this study. Patients undergoing emergency surgery repeated CABG, or undergoing other procedures in addition to CABG were excluded from the study.
Data collection
The baseline characteristics of all participants, including EuroSCORE II values, were obtained from the hospital information system. EuroSCORE II values were calculated at admission. We obtained the preoperative echocardiographic recordings from the local database.
Echocardiographic analysis
Echocardiography was with recommendations from the European Association of Cardiovascular Imaging (EACVI) and the American Society of Echocardiography (ASE), using commercially available systems (11,12). The preoperative echocardiography in this study was transthoracic. Echocardiographic variables include posterior wall thickness at end-diastole (PWTd), left ventricular end-diastolic internal diameter (LVIDd), and interventricular septum thickness at end-diastole (IVSTd). Figure 1 demonstrates the measurement of these parameters. Following the recommendations of the ASE, the LV mass was calculated using the formula LV mass =0.60+ [(PWTd + LVIDd + IVSTd)3 − LVIDd3] ×1.04×0.80. LV mass index (LVMi) was calculated using the formula LVMi =(LV mass)/[body surface area (BSA)], and RWT was calculated using the formula RWT =2× (PWTd)/(LVIDd) (11,13). We used the Du Bois formula to estimate BSA (14). LV hypertrophy was defined as LVMi >95 g/m2 for women and >115 g/m2 for men, and increased RWT was defined as RWT >0.42 (11,13). According to a previous study by Verma et al., we assigned patients into the following 4 groups with LVMi and RWT: normal geometry (normal LVMi and normal RWT), concentric remodeling (normal LVMi and increased RWT), eccentric hypertrophy (LV hypertrophy and normal RWT), and concentric hypertrophy (LV hypertrophy and increased RWT) (15).
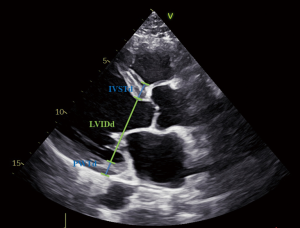
Outcome measures
We recorded the incidence of all-cause death, MI, ischemic stroke, hospitalized heart failure, and resuscitation after cardiac arrest. The primary outcome measures were all-cause death and main adverse cardiovascular and cerebrovascular events (MACCE), and a composite outcome of MI, ischemic stroke, death, hospitalized heart failure and resuscitation after cardiac arrest.
Follow-up
The database was reviewed yearly to identify regular follow-up information. Furthermore, we contacted all participants in this study again by phone using standard forms and procedures.
Statistical analysis
The propensity scores of all patients were established by using a multivariate logistic regression model. The variables in Table 1, except systolic blood pressure and diastolic blood pressure, are the prespecified covariates. Concentric hypertrophy patients were matched with the normal geometry, concentric remodeling, and eccentric hypertrophy patients by using nearest-neighbor propensity score matching. Categorical variables are summarized as percentages, and continuous variables as median (the 25th percentile, the 75th percentile) or mean (± standard deviation). ANOVA or a Kruskal-Wallis test was used to compare continuous variables of more than 2 groups. A Mann-Whitney U test or Student’s t-test was used to compare continuous variables between 2 groups. Fisher’s exact test or χ2 test was used to compare categorical variables. The Kaplan-Meier method and the log-rank test were used to calculate and compare the cumulative survival rate and freedom from MACCE. The independent predictors of MACCE or death were determined by using a Cox proportional hazard model. Variables associated with MACCE or death in the univariate analysis (P<0.1) are included in the multivariate analysis as confounding variables. The area under the curve (AUC), category-free net reclassification improvement (NRI), and integrated discrimination improvement (IDI) were used to evaluate the predictive value of LV geometry for MACCE and death. AUC was calculated by using receiver operating characteristic (ROC) analysis, and NRI and IDI were obtained by using the R package PredictABEL (Suman Kundu, Yurii S. Aulchenko, and A. Cecile J.W. Janssens, 2014). We performed all analyses using R version 3.4.3 and SPSS version 22.0 (IBM, Chicago, Illinois). P values <0.05 were considered significant. A Bonferroni’s test was used for post-hoc comparisons.
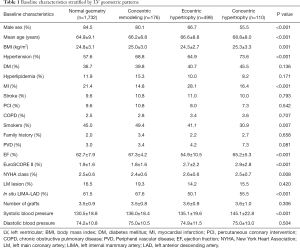
Full table
Results
Patient characteristics
A total of 1,732, 176, 499, 110 patients were assigned into the normal geometry group, the concentric remodeling group, the eccentric hypertrophy group, and the concentric hypertrophy group, respectively. After the propensity score matching (PSM), each group had 110 patients. Figure 2 shows a detailed flow diagram of this study. Patient characteristics before and after PSM are listed in Tables 1 and 2. Before PSM, gender, age, body mass index (BMI), hypertension history, MI history, smoking history, preoperative ejection fraction (EF), EuroSCORE II, New York Heart Association (NYHA) class, in situ left internal mammary artery (LIMA)-left anterior descending coronary artery (LAD), graft completion, and systolic blood pressure was significantly different between the 4 groups (P<0.05) (Table 1). After the PSM, all baseline characteristics, except systolic blood pressure (P=0.003), were similar among the 4 groups (P>0.05) (Table 2).
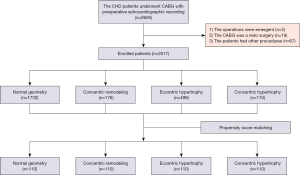
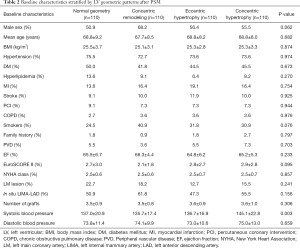
Full table
The relationship between LV geometry and outcomes after CABG
The median follow-up period was 47.0 months (interquartile range, 32.5 to 61.3 months), and the follow-up rate was 93.6%. A total of 74 patients (18.0%) had MACCE events, and 45 (10.9%) died. The patients with concentric hypertrophy had the highest MACCE rate (P<0.001) and mortality (P=0.018) compared to the other 3 groups (Table 3). It was determined that the cumulative freedom from MACCE in concentric hypertrophy (P<0.001) or eccentric hypertrophy (P=0.013) patients was significantly lower than the normal geometry patients (Figure 3). Similarly, the cumulative survival rate in concentric hypertrophy patients was lower compared to the other 3 groups (P=0.006) (Figure 4). The cumulative freedom from MACCE and the cumulative survival rate of the concentric remodeling patients were lower than the normal geometry patients. However, there was no statistical difference (P>0.05) (Figures 3 and 4).
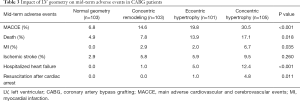
Full table
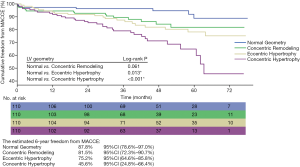
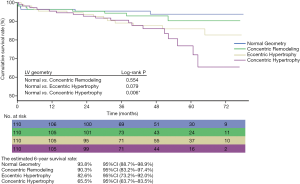
Table 4 shows the results from the Cox proportional hazard models. In univariate analysis, concentric hypertrophy (MACCE: P<0.001; death: P=0.005) and eccentric hypertrophy (MACCE: P=0.017; death: P=0.049) were significant determinants for MACCE or death, however concentric remodeling (MACCE: P=0.069; death: P=0.370) was not statistically different. In multivariate analysis, concentric hypertrophy had a significant association with increased risk of MACCE (P<0.001), as did eccentric hypertrophy (P=0.007) and concentric remodeling (P=0.027). The significant determinants for death were eccentric hypertrophy (P=0.024) and concentric hypertrophy (P=0.033). LVMi (MACCE: P=0.004; death: P=0.014) and RWT (MACCE: P=0.001; death: P=0.007) as continuous variables are significantly associated with an increased risk of MACCE or death in multivariate analysis. Systolic blood pressure and diastolic blood pressure are not significant determinants for MACCE or death (P>0.05).
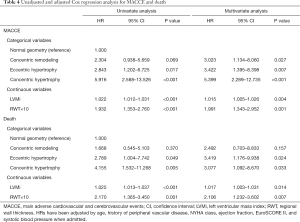
Full table
Incremental prognostic value of LV geometry over EuroSCORE II
Adding LV geometry to EuroSCORE II did not significantly increase the AUC to predict MACCE (from 0.621 to 0.696; P=0.054) or death (from 0.648 to 0.691; P=0.218) (Table 5). After adding LVMi and RWT to EuroSCORE II as continuous variables, the AUC for MACCE increased significantly (from 0.621 to 0.703; P=0.042), but not for death (from 0.648 to 0.713; P=0.134) (Table 5). Reclassification measures (NRI and IDI) for MACCE or death improved significantly after incorporating either LVMi and RWT or LV geometry (Table 5). Compared with EuroSCORE II, the addition of LV geometry resulted in an IDI of 0.043 for MACCE (P<0.001) and 0.018 for death (P=0.020), which indicated an absolute increase of 4.3% in mean MACCE risk and 1.8% in mean death risk for patients with events compared to patients without events over EuroSCORE II. The addition of LVMi and RWT resulted in an IDI of 0.068 for MACCE (P<0.001) and 0.060 for death (P=0.001). The new model, including LV geometry, had an NRI of 0.200 for MACCE (P<0.001) and 0.308 for death (P=0.002). Moreover, the model, including LVMi and RWT, had an NRI of 0.212 for MACCE (P<0.001) and 0.256 for death (P=0.043).
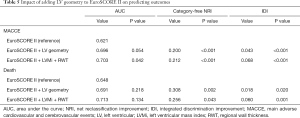
Full table
Discussion
The results of this study confirmed that LV geometry was an independent prognostic factor for MACCE or death in patients undergoing CABG. Furthermore, the predictive ability of MACCE or death in CABG patients was improved significantly by incorporating LVMi and RWT or LV geometry with EuroSCORE II.
Increased LV mass and abnormal LV geometric patterns, including concentric ventricular remodeling, eccentric hypertrophy, and concentric hypertrophy, result from volume and pressure overload (6,15). They are considered to reflect the chronicity and severity of cardiovascular risk factors and may have better prognostic ability than current predictors (16,17). The prognostic implications of LV mass and geometry have been confirmed in populations with different cardiac diseases, including CHD, left ventricular systolic dysfunction and aortic stenosis (18-20). However, clinical data on the prognostic value of LV geometry in CABG patients is lacking. CABG is an essential treatment for CHD. CABG patients usually have severe CHD, and an increasing proportion of patients referred for CABG have severe complications and LV geometric adaptations. Since LV geometry has predictive value for CHD prognosis, there may be predictive potential for CABG patients.
This study demonstrated a significant association between abnormal LV geometry and cardiovascular risk. According to the multivariate Cox regression analyses, all LV geometric adaptations are independent risk factors for MACCE, and concentric LV hypertrophy has the highest risk of adverse events, therefore confirming the prognostic value of LV geometry in CABG patients. The renin-angiotensin-aldosterone system and hypertension play essential roles in the progression of LV hypertrophy and CHD. Increased LV mass that exceeds the scope of compensation for the increased cardiac load is a pathological process found in concentric LV hypertrophy (21,22). Moreover, there is abnormal collagen deposition around the intramyocardial coronary arteries and the extracellular matrix in patients with pathological increases in LV mass, inducing medial thickening, and stenosis of coronary arteries (23,24). For CABG patients, the extracellular collagen deposition is also associated with the calcification and failure of vein graft (25). LV hypertrophy induces disorganization of the sarcomere, loss of myocytes with fibrotic replacement, changes in glycolytic metabolism, alterations in calcium handling, adaptations in contractility, diastolic and systolic dysfunction, and electrical remodeling (26). These changes in structure, metabolism, and function may facilitate the association between LV hypertrophy and graft lesions, heart failure, or adverse cardiovascular events.
The rapidly growing use of PCI for myocardial revascularization and the aging of the population has led to many patients with severe complications, and abnormal LV geometry refers to CABG, which increases the risk of adverse events. Hence, better risk stratification and tailored treatment strategies are needed. There have been some evaluation systems used to predict the risk of perioperative and long-term adverse events in patients undergoing cardiac surgery, including EuroSCORE and EuroSCORE II (27-31). None of these evaluation systems incorporate information about LV geometry, despite being significantly associated with adverse events after CABG, as observed in this study. We demonstrated that LV mass or geometry provided an incremental prognostic value EuroSCORE II to predict mid-term MACCE or death in patients after CABG. Given that echocardiography is one of the routine tests before CABG, echocardiographic based LV geometry assessment can, therefore, be a practical and useful clinical tool for improving risk prediction and risk management in CABG patients.
This initial analysis of LV mass and geometry focused on individuals undergoing CABG. There is an additional need for accurate cardiovascular risk prediction for other cardiac interventions. Furthermore, this study is non-randomized, and another potential limitation of this study is that there might still be residual confounders. Moreover, patients without echocardiographic measurements at admission were not included in this study, which might have introduced selection bias.
Conclusions
LV geometry was an independent and incremental prognostic factor for MACCE and death in CABG patients.
Acknowledgments
We thank Dr. Yuehua Fang for the valuable discussion.
Funding: This paper was supported by the Cultivation Program from Ruijin Hospital for Youth Program of National Natural Science Foundation (2019QNPY02021).
Footnote
Conflicts of Interest: All authors have completed the ICMJE uniform disclosure form (available at http://dx.doi.org/10.21037/qims-19-926). The authors have no conflicts of interest to declare.
Ethical Statement: The institutional ethics board approved the study of Rui Jin Hospital (NO. 2018-15), and informed consent was taken from all the patients.
Open Access Statement: This is an Open Access article distributed in accordance with the Creative Commons Attribution-NonCommercial-NoDerivs 4.0 International License (CC BY-NC-ND 4.0), which permits the non-commercial replication and distribution of the article with the strict proviso that no changes or edits are made and the original work is properly cited (including links to both the formal publication through the relevant DOI and the license). See: https://creativecommons.org/licenses/by-nc-nd/4.0/.
References
- Benjamin EJ, Blaha MJ, Chiuve SE, Cushman M, Das SR, Deo R, de Ferranti SD, Floyd J, Fornage M, Gillespie C, Isasi CR, Jimenez MC, Jordan LC, Judd SE, Lackland D, Lichtman JH, Lisabeth L, Liu S, Longenecker CT, Mackey RH, Matsushita K, Mozaffarian D, Mussolino ME, Nasir K, Neumar RW, Palaniappan L, Pandey DK, Thiagarajan RR, Reeves MJ, Ritchey M, Rodriguez CJ, Roth GA, Rosamond WD, Sasson C, Towfighi A, Tsao CW, Turner MB, Virani SS, Voeks JH, Willey JZ, Wilkins JT, Wu JH, Alger HM, Wong SS, Muntner P. American Heart Association Statistics C, Stroke Statistics S. Heart Disease and Stroke Statistics-2017 Update: A Report From the American Heart Association. Circulation 2017;135:e146-e603. [Crossref] [PubMed]
- Gaudron P, Eilles C, Kugler I, Ertl G. Progressive left ventricular dysfunction and remodeling after myocardial infarction. Potential mechanisms and early predictors. Circulation 1993;87:755-63. [Crossref] [PubMed]
- Rumberger JA, Behrenbeck T, Breen JR, Reed JE, Gersh BJ. Nonparallel changes in global left ventricular chamber volume and muscle mass during the first year after transmural myocardial infarction in humans. J Am Coll Cardiol 1993;21:673-82. [Crossref] [PubMed]
- Kovacs Z, Kormanyos A, Domsik P, Kalapos A, Lengyel C, Ambrus N, Ajtay Z, Piros GA, Forster T, Nemes A. Left ventricular longitudinal strain is associated with mitral annular fractional area change in healthy subjects-Results from the three-dimensional speckle tracking echocardiographic MAGYAR-Healthy Study. Quant Imaging Med Surg 2019;9:304-11. [Crossref] [PubMed]
- Chu M, Qian L, Zhu M, Yao J, Xu D, Chen M. Circumferential strain rate to detect lipopolysaccharide-induced cardiac dysfunction: a speckle tracking echocardiography study. Quant Imaging Med Surg 2019;9:151-9. [Crossref] [PubMed]
- Vakili BA, Okin PM, Devereux RB. Prognostic implications of left ventricular hypertrophy. Am Heart J 2001;141:334-41. [Crossref] [PubMed]
- Levy D, Garrison RJ, Savage DD, Kannel WB, Castelli WP. Prognostic implications of echocardiographically determined left ventricular mass in the Framingham Heart Study. N Engl J Med 1990;322:1561-6. [Crossref] [PubMed]
- Ghali JK, Kadakia S, Cooper RS, Liao YL. Impact of left ventricular hypertrophy on ventricular arrhythmias in the absence of coronary artery disease. J Am Coll Cardiol 1991;17:1277-82. [Crossref] [PubMed]
- Nashef SA, Roques F, Sharples LD, Nilsson J, Smith C, Goldstone AR, Lockowandt U. EuroSCORE II. Eur J Cardiothorac Surg 2012;41:734-44; discussion 744-5. [Crossref] [PubMed]
- Krumholz HM, Larson M, Levy D. Prognosis of left ventricular geometric patterns in the Framingham Heart Study. J Am Coll Cardiol 1995;25:879-84. [Crossref] [PubMed]
- Lang RM, Badano LP, Mor-Avi V, Afilalo J, Armstrong A, Ernande L, Flachskampf FA, Foster E, Goldstein SA, Kuznetsova T, Lancellotti P, Muraru D, Picard MH, Rietzschel ER, Rudski L, Spencer KT, Tsang W, Voigt JU. Recommendations for cardiac chamber quantification by echocardiography in adults: an update from the American Society of Echocardiography and the European Association of Cardiovascular Imaging. J Am Soc Echocardiogr 2015;28:1-39.e14. [Crossref] [PubMed]
- Choi JO, Shin MS, Kim MJ, Jung HO, Park JR, Sohn IS, Kim H, Park SM, Yoo NJ, Choi JH, Kim HK, Cho GY, Lee MR, Park JS, Shim CY, Kim DH, Shin DH, Shin GJ, Shin SH, Kim KH, Park JH, Lee SY, Kim WS, Park SW. Normal Echocardiographic Measurements in a Korean Population Study: Part I. Cardiac Chamber and Great Artery Evaluation. J Cardiovasc Ultrasound 2015;23:158-72. [Crossref] [PubMed]
- Marwick TH, Gillebert TC, Aurigemma G, Chirinos J, Derumeaux G, Galderisi M, Gottdiener J, Haluska B, Ofili E, Segers P, Senior R, Tapp RJ, Zamorano JL. Recommendations on the Use of Echocardiography in Adult Hypertension: A Report from the European Association of Cardiovascular Imaging (EACVI) and the American Society of Echocardiography (ASE). J Am Soc Echocardiogr 2015;28:727-54. [Crossref] [PubMed]
- Du Bois D, Du Bois EF. A formula to estimate the approximate surface area if height and weight be known. 1916. Nutrition 1989;5:303-11; discussion 312-3. [PubMed]
- Verma A, Meris A, Skali H, Ghali JK, Arnold JM, Bourgoun M, Velazquez EJ, McMurray JJ, Kober L, Pfeffer MA, Califf RM, Solomon SD. Prognostic implications of left ventricular mass and geometry following myocardial infarction: the VALIANT (VALsartan In Acute myocardial iNfarcTion) Echocardiographic Study. JACC Cardiovasc Imaging 2008;1:582-91. [Crossref] [PubMed]
- Verdecchia P, Schillaci G, Borgioni C, Ciucci A, Battistelli M, Bartoccini C, Santucci A, Santucci C, Reboldi G, Porcellati C. Adverse prognostic significance of concentric remodeling of the left ventricle in hypertensive patients with normal left ventricular mass. J Am Coll Cardiol 1995;25:871-8. [Crossref] [PubMed]
- Milani RV, Lavie CJ, Mehra MR, Ventura HO, Kurtz JD, Messerli FH. Left ventricular geometry and survival in patients with normal left ventricular ejection fraction. Am J Cardiol 2006;97:959-63. [Crossref] [PubMed]
- Bohbot Y, Rusinaru D, Delpierre Q, Marechaux S, Tribouilloy C. Impact of Preoperative Left Ventricular Remodelling Patterns on Long-Term Outcome after Aortic Valve Replacement for Severe Aortic Stenosis. Cardiology 2018;139:105-9. [Crossref] [PubMed]
- Ghali JK, Liao Y, Cooper RS. Influence of left ventricular geometric patterns on prognosis in patients with or without coronary artery disease. J Am Coll Cardiol 1998;31:1635-40. [Crossref] [PubMed]
- Nakamori S, Ismail H, Ngo LH, Manning WJ, Nezafat R. Left ventricular geometry predicts ventricular tachyarrhythmia in patients with left ventricular systolic dysfunction: a comprehensive cardiovascular magnetic resonance study. J Cardiovasc Magn Reson 2017;19:79. [Crossref] [PubMed]
- de Simone G, Verdecchia P, Pede S, Gorini M, Maggioni AP. Prognosis of inappropriate left ventricular mass in hypertension: the MAVI Study. Hypertension 2002;40:470-6. [Crossref] [PubMed]
- Mureddu GF, Pasanisi F, Palmieri V, Celentano A, Contaldo F, de Simone G. Appropriate or inappropriate left ventricular mass in the presence or absence of prognostically adverse left ventricular hypertrophy. J Hypertens 2001;19:1113-9. [Crossref] [PubMed]
- Weber KT, Brilla CG. Pathological hypertrophy and cardiac interstitium. Fibrosis and renin-angiotensin-aldosterone system. Circulation 1991;83:1849-65. [Crossref] [PubMed]
- Weber KT, Janicki JS, Shroff SG, Pick R, Chen RM, Bashey RI. Collagen remodeling of the pressure-overloaded, hypertrophied nonhuman primate myocardium. Circ Res 1988;62:757-65. [Crossref] [PubMed]
- Pedigo SL, Guth CM, Hocking KM, Banathy A, Li FD, Cheung-Flynn J, Brophy CM, Guzman RJ, Komalavilas P. Calcification of Human Saphenous Vein Associated with Endothelial Dysfunction: A Pilot Histopathophysiological and Demographical Study. Front Surg 2017;4:6. [Crossref] [PubMed]
- Hill JA, Olson EN. Cardiac plasticity. N Engl J Med 2008;358:1370-80. [Crossref] [PubMed]
- Kofler M, Reinstadler SJ, Stastny L, Dumfarth J, Reindl M, Wachter K, Rustenbach CJ, Muller S, Feuchtner G, Friedrich G, Metzler B, Grimm M, Bonaros N, Baumbach H. EuroSCORE II and the STS score are more accurate in transapical than in transfemoral transcatheter aortic valve implantation. Interact Cardiovasc Thorac Surg 2018;26:413-9. [Crossref] [PubMed]
- Singh N, Gimpel D, Parkinson G, Conaglen P, Meikle F, Lin Z, Kejriwal N, Odom N, McCormack DJ, El-Gamel A. Assessment of the EuroSCORE II in a New Zealand Tertiary Centre. Heart Lung Circ 2019;28:1670-6. [Crossref] [PubMed]
- Ben Ahmed H, Chelli M, Selmi K, Bouzouita K, Mokaddem A, Ben Ameur Y, Boujnah MR. Single versus bilateral internal thoracic artery grafts for multi vessel coronary artery bypass grafting: mid-term results. Tunis Med 2012;90:720-4. [PubMed]
- Chung WJ, Chen CY, Lee FY, Wu CC, Hsueh SK, Lin CJ, Hang CL, Wu CJ, Cheng CI. Validation of Scoring Systems That Predict Outcomes in Patients With Coronary Artery Disease Undergoing Coronary Artery Bypass Grafting Surgery. Medicine (Baltimore) 2015;94:e927. [Crossref] [PubMed]
- Hansen LS, Hjortdal VE, Andreasen JJ, Mortensen PE, Jakobsen CJ. 30-day mortality after coronary artery bypass grafting and valve surgery has greatly improved over the last decade, but the 1-year mortality remains constant. Ann Card Anaesth 2015;18:138-42. [Crossref] [PubMed]