Neuroimaging endophenotypes of type 2 diabetes mellitus: a discordant sibling pair study
Introduction
As a chronic metabolic disease, the cause of type 2 diabetes mellitus (T2DM) is considered to be a mixture of genetic and epigenetic predispositions interacting with complex societal factors (1). Although T2DM is mostly driven by lifestyle changes, heredity is also considered to be very important in determining its risk (2). Noticeable familial aggregation is one of the features of T2DM. The heritability is approximately 70–80%, and a high prevalence in the siblings of affected individuals has been observed compared to that of the general population, with a ratio of 3.5–4 for T2DM (2-4).
In recent decades, at least 75 independent genetic loci have been found for T2DM, which allows us to better understand the genetic architecture of T2DM (5). In addition, a few of genetic polymorphisms and variants have been proven to be associated with the T2DM-related cognitive impairment and cerebral abnormalities. MYH9 and NLRX1 gene polymorphisms were found to be associated with the susceptibility of T2DM cerebrovascular abnormalities (6,7). Coding variants of CNST, PLAA, and PCDH8 were found to be associated with cognitive performance in family-based T2DM-enriched populations (8), and, in the same populations, a missense variant in PLEKHG4B from the exome-wide array was discovered to be significantly associated with neuroimaging measures of white matter (WM) mean diffusivity and grey matter (GM) mean diffusivity in T2DM (9). The identification of a heritable component to cognitive performance and neuroimaging measures in T2DM suggests the role of genetic contributors in the related cerebral abnormalities. However, identifying these causal variants and genes by classic linkage and association designs is still challenging (5,10). Furthermore, it is argued that traditional phenotypes widely used in the clinic may involve considerable genetic heterogeneity and are too far “downstream” from the sites of the working gene to support a powerful and replicable linkage to specific variations. Under these circumstances, interest has been stimulated in the study of endophenotypes, which are also known as intermediate phenotypes.
Endophenotypes are heritable and quantitative markers associated with illness liability; they must be independent of the clinical state (manifest in illness whether it is active or not) and co-segregate with the illness within a family; they can be found both in the probands and unaffected relatives at a higher rate compared with the general population (11,12). The study of endophenotypes is believed to be an important step towards understanding heritable risk factors and is useful in unravelling the genetic basis of diseases. In addition, as evidenced by the abundant publications on endophenotype markers in psychiatric diseases, endophenotypes seem also to be relevant in medical illnesses, including T2DM, hypertension, and familial hypercholesterolemia (12).
Neuroimaging endophenotypes are considered to be valuable in the analysis of genetic liability for an illness owing to their good repeatability and sensitivity of quantitative data (13). Neuroimaging methods have been widely used in neurological disorders related to T2DM and have provided important insights into the pathophysiology of T2DM. Indeed, previous studies have not only revealed that T2DM is associated with brain abnormalities and cognition decline, but have also suggested that neurological disorders, such as emotional eating, obesity, and insulin resistance, participate in the pathogenesis of T2DM (14-16). Furthermore, Cox’s and Raffield’s studies are evidence that, in populations with T2DM, genetic factors contribute to variants of neuroimaging measurements and cognitive performance, respectively (8,9). Therefore, it is important to consider the complex genetic factors in the effects of T2DM-related neurological disorders and verify the earliest neuro-signs of impending T2DM. However, to the best of our knowledge, few studies have focused on the neuroimaging endophenotypes of T2DM.
In this study, we conducted sibling pair designs in which endophenotypes were verified with reduced variability in related pairs, including one proband and one genetically unaffected relative. Capitalizing on voxel-based morphometry (VBM) and three-dimensional pseudo-continuous arterial spin labelling (3D-pCASL), we hypothesized that the unaffected siblings of T2DM patients would share overlapped brain regions of abnormal GM density and cerebral blood flow (CBF) with T2DM patients compared with unrelated controls.
Methods
Subjects
This study was approved by the ethical review committee of the First Affiliated Hospital of Jinan University (Guangzhou, China), and written informed consent was obtained. A group of patients with T2DM and their unaffected first-degree relatives (siblings) were recruited for this study, along with unrelated controls. The T2DM patients met the criteria recommended by the American Diabetes Association in 2010 (17), and were confirmed by diabetologists in clinic and diagnosed with diabetes for more than 2 years. The age gap between the T2DM patients and their unaffected siblings was no more than 5 years. The unaffected siblings and unrelated controls were confirmed by diabetologists based on a routine medical examination within 6 months, and an additional measurement of a fasting finger-prick blood glucose test of less than 7.0 mmol/L was performed to verify the absence of diabetes. All the subjects were right-handed and had at least 6 years of education.
The exclusion criteria for all subjects were a history of stroke, drug or alcohol dependence, head trauma, epilepsy, Parkinson’s disease, neuropsychiatric disorder, severe visual or hearing loss, and any contraindications to MRI. Subjects with a major medical illness (e.g., cancer, anaemia, diabetic ketoacidosis, thyroid dysfunction, and chronic nephropathy) and a history of severe hypoglycaemia attacks were also excluded from the present study. Moreover, the evaluation of WM hyperintensity was performed on the fluid-attenuated inversion recovery (FLAIR) images with the age-related WM changes (ARWMC) scale (18) by two experienced radiologists who were blinded for the clinical data and group allocation; participants with a rating score above 1 were excluded (19). Consensus was achieved through discussion between the two observers. We further excluded any unrelated controls with a first-degree relative with T2DM or psychotic disorder. Eventually, of the 90 recruited subjects, 26 pairs of T2DM patients and their unaffected siblings were screened for the subsequent study, along with 26 unrelated controls matched by age, sex, and education level (Figure 1).
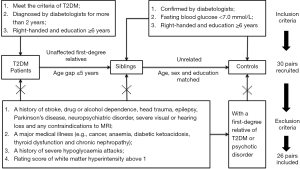
Neuropsychological tests
The cognitive status of the subjects was assessed by a battery of neuropsychological tests covering major cognitive domains. The Mini-Mental State Exam (MMSE) and Montreal Cognitive Assessment (MoCA) tests were performed to evaluate the general level of cognition. The Clock Drawing Test (CDT) and Rey-Osterrieth Complex Figure (ROCF) test were used to measure the visual-spatial ability and visual memory function, including immediate recall and delayed recall tests. In addition, Logical Memory II (Immediate and Delayed Recall tests) from the Wechsler Memory Scale-Revised, the Prospective and Retrospective Memory Questionnaire (PRMQ), the Comprehensive Assessment of Prospective Memory (CAPM), and the Instrumental Activities of Daily Living scale (IADL) were also used in the current study.
MRI acquisition
Imaging data were acquired using a 3.0 T MRI system (MR750, GE Healthcare, Milwaukee, WI, USA) with an 8-channel head coil array. Conventional brain imaging, including T1-weighted image (T1WI), T2-weighted image (T2WI), and FLAIR, was obtained and assessed by two experienced radiologists to exclude serious brain diseases.
Structural MRI
An axial 3D brain volume imaging (3D-BRAVO) was acquired for T1WI with the following parameters: echo time (TE) =3.1 ms, repetition time (TR) =7.9 ms, field of view (FOV) =240×240 mm2, matrix =240×240, slice thickness =1 mm, slice number =160, bandwidth =31.25 kHz, and number of excitations (NEX) =1. The whole procedure required 3 min 47 s.
3D-pCASL
The 3D-pCASL scans were performed using background-suppressed and a stack-of-spirals 3D-fast-spin echo imaging sequences with the following parameters: post label delay (PLD) =1,525 ms, TR/TE =4,890/11.1 ms, FOV =240×240 mm2, matrix =512×8, section thickness =3.0 mm, slice number =52, bandwidth =62.5 kHz, and NEX =3. The whole procedure required 4 min 44 s.
Image analyses
Preprocessing was conducted blind to age, sex, diabetes status, and clinical or cognitive measurements.
Structural MRI analyses
The VBM analysis was performed on the structural T1W images using the VBM8 (http://dbm.neuro.uni-jena.de/vbm8/) toolbox for Statistical Parametric Mapping 8 (SPM8, http://www.fil.ion.ucl.ac.uk/spm/software/spm8/). For each subject, the high-resolution structural image was segmented into GM, WM, and cerebrospinal fluid (CSF) components. Then, the GM maps were normalized to the MNI standard space by using the high-dimensional diffeomorphic anatomical registration through exponentiated lie algebra (DARTEL) template. Modulated spatial normalized GM image smoothing with a full width at half maximum (FWHM) Gaussian kernel of 8 mm was used to account for the effect of brain volumes.
3D-pCASL analyses
The CBF maps of each participant were obtained offline using an independent workstation (Advantage Workstation 4.5, GE Healthcare).
Statistical analysis
Demographic, laboratory and cognitive data were analysed using SPSS (v. 14, Chicago, IL, USA). One-way analysis of variance and χ2 test were used to explore differences in quantitative data and categorical variables among the three groups (T2DM patients, unaffected siblings, and unrelated controls). A P value less than 0.05 was deemed statistically significant.
The neuroimaging statistical analysis was implemented by using the Resting-State fMRI Data Analysis Toolkit (20). A two-sample Student’s t-test was performed to assess the between-group differences with the relative volume of the brain, age, and gender as covariates. For all statistical analysis results, Monte Carlo simulation was applied to revise the multiple comparisons. We conducted the correction with the AlphaSim program in “REST” with the following parameters: FWHM =8 mm, cluster connection radius r =5 mm, single-voxel P threshold =0.01, iterations =1,000. A threshold of P<0.01 and a minimum cluster size of 385 voxels were used for minimizing the false positives and counting the significant clusters within the group.
Results
Demographic and clinical data
The sample characteristics are provided in Table 1, and three groups were well-matched in terms of age, gender, education level, and body mass index (BMI). T2DM patients scored poorer in MoCA than unrelated controls (P=0.012), and no significant differences were observed among the three groups in the other neuropsychological tests.
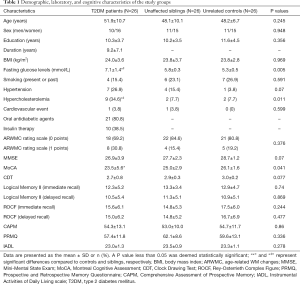
Full table
Comparison of cerebral GM volume across groups
Compared with unrelated controls, T2DM patients showed significant atrophy in the right middle frontal cortex, right inferior temporal cortex, right middle temporal cortex, right supramarginal cortex, bilateral insular and opercular cortex, left precuneus, bilateral cingulate cortex, and bilateral orbitofrontal cortex (Table 2, Figure 2).
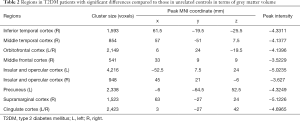
Full table
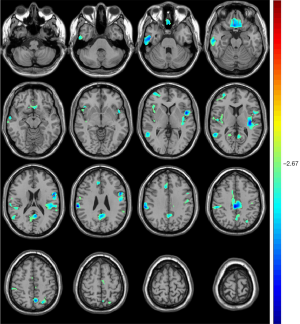
Compared with unrelated controls, the unaffected siblings showed significant atrophy of the right middle/inferior temporal cortex and left insular cortex along with significant expansion of the right cuneus/calcarine (Table 3, Figure 3).
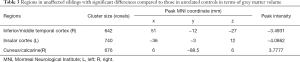
Full table
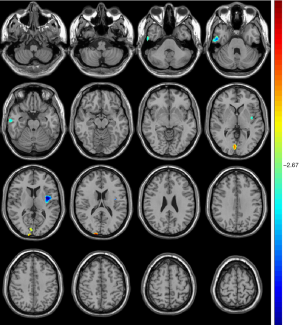
Comparison of CBF across groups
Compared with unrelated controls, T2DM patients presented significantly increased CBF in the right temporopolar, right superior frontal, and right middle frontal gyrus (Table 4, Figure 4).
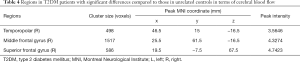
Full table
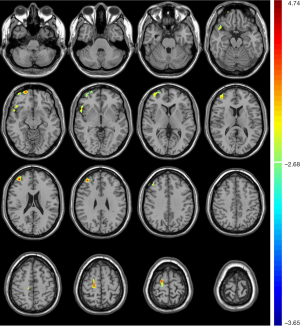
Compared with unrelated controls, the unaffected siblings presented significantly decreased CBF in the right mesial temporal lobe, right inferior/middle temporal gyrus, left middle temporal gyrus, left insula and operculum, and right supramarginal gyrus, in addition to increased CBF in the right lingual gyrus, bilateral middle frontal gyrus, right superior frontal gyrus, and bilateral anterior cingulate cortex (Table 5, Figure 5).
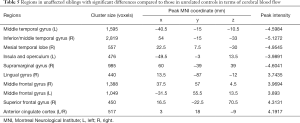
Full table
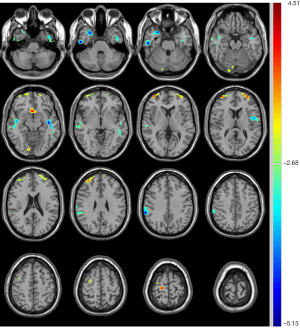
Discussion
Based on the familial aggregation in T2DM and the observed high prevalence in the siblings of affected individuals, we designed a sibling pair study, attempted to verify the neuroimaging endophenotypes of T2DM, and explored the effects of complex genetic factors of T2DM in brain abnormalities from structural and functional MRI. We observed extensive brain abnormalities in T2DM patients compared with unrelated controls. We also verified the hypothesis that unaffected siblings have specific overlapping brain regions of abnormal GM volume and CBF with T2DM patients.
Cognitive status in T2DM patients and the siblings
In our study, several neuropsychological tests were performed to evaluate the cognitive status of the subjects, and only the MoCA test showed significant difference between the T2DM patients and unrelated controls, while MMSE did not. MMSE and MoCA are the most frequently used omnibus tests for office-based assessment of cognition. However, MoCA covers more cognitive domains and has greater speciality and sensitivity compared with MMSE, especially for the detection of mild cognitive impairment (MCI) (21,22). Furthermore, MoCA includes a minor correction for lower educational levels, while MMSE does not, which affects the diagnostic accuracy of the latter in highly educated individuals (23). According to the results of the neuropsychological tests in the current study, T2DM patients presented a decreased cognitive status compared with unrelated controls, but the unaffected siblings did not. However, the assessment of cognitive status using mental scales is limited by the subjectivity of examiners and the subjects, and further objective neuroimaging evidence is required.
Abnormal GM volume in T2DM patients and their siblings
According to our VBM analysis, T2DM patients presented extensive atrophy of brain regions mainly involving the right inferior and middle temporal cortex, right prefrontal cortex, right supramarginal cortex, left precuneus, bilateral insular and opercular cortex, bilateral cingulate cortex, and bilateral orbitofrontal cortex, which suggests significant neuronal loss in the brain. Diabetic glucose disorders and deterioration in glucose metabolism are thought to be associated with neuronal loss and the decline of brain volumes (24), along with insulin resistance and decreased insulin receptor expression in the brain, impaired insulin signalling, and reduced insulin levels in cerebrospinal fluid (16). The distribution of brain atrophy our study revealed in T2DM has been identified by several previous studies (19,25,26). However, the medial temporal lobe showed no significant atrophy in our results, especially in the region of the hippocampus which had been found most pronounced in T2DM-related brain atrophy (27,28). The absence of atrophy in the medial temporal lobe also appeared in Chen’s research, who used VBM to investigate the pattern of brain volume changes of the brain in T2DM patients (mean age 61.2 and mean MMSE score 26.0). Meanwhile, in Zhang’s study, atrophy of the hippocampus and parahippocampus only appeared in T2DM patients with MCI (mean age 56.2 and mean MoCA score 16.4) and disappeared in T2DM patients without MCI (mean age 52.2 and mean MoCA score 26.7) (25). These inconsistent results may be because the T2DM patients we included in the present study were younger (mean age 51.9) and had better cognitive status (mean MMSE/MoCA score 26.9/23.5) than patients in other studies. In addition, the results of a pooled analysis revealed that T2DM patients had greater brain but not hippocampal atrophy compared with controls, and their findings do not support the specific vulnerability of the hippocampus in T2DM patients (29).
For the unaffected siblings of T2DM patients, there is significant shared atrophy only in the regions of the right inferior/middle temporal and left insula cortex with T2DM patients when compared to that of controls. The GM atrophy in the right temporal lobe and left rolandic operculum region was emphasized in Chen’s research on T2DM-associated cerebral structural mapping using VBM and was attributed to the cortical microinfarcts to some extent (19). The atrophy of the left insula in T2DM patients has also been reported by Moran (26). However, the significant atrophy of the temporal lobe and insula in unaffected siblings of T2DM patients was first reported by us in the current study, and these regions were supposed to be associated with changes in glucose metabolism, adiponectin, and insulin resistance, and may participate in the pathogenesis of T2DM (30-32). It is worth noting that the atrophy of the left insula in our study was mainly located at the posterior regions. The left insula revealed significantly greater activity in response to the tastant through gustatory mapping task, and the posterior insula was found to be the taste-sensitive region, was directly related to the levels of peripheral glucose, played an important role in the category-specific integration of homeostatic signals, and participated in the process that maintains and regulates the body’s homeostatic state (33). Accordingly, the atrophy of the left insula in both T2DM patients and their unaffected siblings may represent a disorder in the body’s homeostatic state and may participate in the pathogenesis of initial T2DM.
Abnormal CBF in T2DM patients and siblings
Perfusion is an important parameter to estimate dysfunction in the level of brain tissues (34). Arterial spin labelling (ASL) has emerged as a useful tool for CBF evaluation in clinical practice because of its noninvasive, nonradioactive, and nonpoisonous advantages (35). Correcting for brain atrophy, our results showed no significant decrease in CBF in T2DM patients; instead, there was a significant increase mainly in the right prefrontal lobe compared with controls. Studies on the changes in CBF in T2DM patients showed conflicting results (36). Last’s study on T2DM patients (mean age 61.6) with ASL-MRI showed reduced CBF in all brain regions (37). However, using the same method, Rusinek’s research presented no significant hypoperfusion in T2DM patients (mean age 54.2) (38). Even though the difference in the age and cognitive status of included participants among them may contribute to the conflicting results, it should be noted that not all studies corrected their CBF measures for brain atrophy (36). The reported hypoperfusion in T2DM patients might be an “artefact” due to cerebral atrophy, and significant reductions in CBF did not remain significant when corrected for atrophy (39).
Interestingly, the siblings not only shared the overlapped elevated CBF region of the right prefrontal lobe with T2DM patients but also presented more decreased regions, mainly involving the right inferior/middle temporal gyrus, right mesial temporal lobe, right supramarginal gyrus, left insula and operculum, in addition to other increased regions of the left prefrontal lobe, bilateral anterior cingulate cortex, and right lingual gyrus, when compared to controls. The controversial results also appeared in Rusinek’s research on patients with insulin resistance and T2DM, and the positive treatment effect of blood glucose, cholesterol, and BP lowering medications was considered to account for it (38). As for the frontal lobe, an increased CBF observed in our study may reflect an activity neuronal function accompanied with an alteration in membrane or myelin turnover, which was proven by MRS in patients with metabolic syndrome (40). The prefrontal lobe is likewise involved in reward processing and decision making (41). An elevated CBF in the prefrontal lobe revealed in siblings and T2DM patients may be associated with the abnormal activation of food reward and may lead to subsequent anomalous overeating and obesity, which have been regarded as risk factors for T2DM (41,42). Notably, most regions of abnormal CBF in siblings overlapped with atrophy regions observed in T2DM patients compared to controls, including the right inferior/middle temporal gyrus, left insula and operculum, right supramarginal gyrus, right prefrontal lobe, and bilateral anterior cingulate cortex. The variation of these regions from function to structure may indicate an important role of central nervous system disorders in the deterioration process of T2DM, and the neurodegeneration caused by heritable components may precede the occurrence of T2DM.
Candidacy and utility of neuroimaging endophenotypes for T2DM
Previous studies have proposed that endophenotypes are quantitative heritable traits that are abnormal in both probands and their relatives (11,12). Neuroimaging markers found in our research were quantitatively abnormal, with significant differences in both T2DM patients and unaffected siblings compared to populations. These markers included atrophy in the right inferior/middle temporal gyrus and left insula and elevated perfusion in the right prefrontal lobe. A twin-pair study may demonstrate strict sense heritability, but a higher concordance rate between twins compared to a sibling pair may partly be due to shared environmental factors, such as the intrauterine environment (43). In addition, familial heritability (rather than strict sense) is estimated to be 70–80% (4); hence, it is practical and feasible for sibling pair designs to study the endophenotypes of T2DM (8,9).
The study of neuroimaging endophenotype-based sibling pair designs has the immediate advantage of discounting any non-familial explanations for abnormal patterns of brain structure, such as the exposure to medication in the probands (44). Endophenotypes could help to refine the subclassification of patients based on the extent of expressed endophenotypic abnormality and be used to screen the high-risk populations for T2DM. Furthermore, our results might be exploited in the future to identify specific genes determining variation in the central nervous system that are important for the pathogenesis of T2DM. In principle, the neuroimaging endophenotypes could be used as quantitative traits in a genome-wide search for associated polymorphisms by quantitative trait locus (QTL) analysis, and this method has been successfully used by Beatty et al. to identify genetic markers associated with imaging measurements of GM volumes in inbred strains of mice (45).
There are several limitations in our study that may be addressed in future work. First, the relatively small sample size restricted us to perform the subgroup analysis of the siblings according to the level of insulin resistance or glucose tolerance, and it would be more interesting to use a large sample size in the future. Second, this is a cross-sectional study, and the causal links cannot be proven; hence, a longitudinal study aimed at unaffected siblings would be necessary to investigate the developmental difference in the neural mechanism of T2DM from function to structure. Third, most T2DM patients included in our study received medication therapy, which might have affected the results of CBF. Therefore, the issue of medication needs to be addressed in the future by adding samples of drug-naïve patients with T2DM.
Conclusions
Capitalizing on neuroimaging methods, we conducted a sibling pair study to investigate the related cerebral functional and structural endophenotypes of heritable but genetically complex T2DM. Our results found that the unaffected siblings shared significant GM loss in the right inferior/middle temporal gyrus and left insula along with elevated perfusion in the right prefrontal lobe with T2DM patients compared to controls. These results might serve as the neuroimaging endophenotypes for T2DM in the brain according to the definition of endophenotypes. Our findings also revealed that several cerebral regions of abnormal CBF in siblings overlapped with atrophy observed in T2DM patients, which indicates the increased risk for T2DM, and the variation of these regions from function to structure may underlie the heritable neurodegeneration of T2DM in the brain.
Acknowledgments
Funding: This study is supported by grants from the Guangzhou Key Laboratory of Molecular and Functional Imaging for Clinical Translation (Project No. 201905010003), the Engineering Research Center of Medical Imaging Artificial Intelligence for Precision Diagnosis and Treatment, Guangdong Province, the Collaborative Innovation Program of Hong Kong and Guangdong Province, the Guangdong Science and Technique Department (Project No. 2016A050503032), the Guangzhou External Science and Technology Cooperation Program (Project No. 201807010046), the Shenzhen Science and Technology Innovation Committee (Project No. CYZZ20160421160735632), the Research Grants Council of the Hong Kong Special Administrative Region, China (Project No. CUHK 14113214 and CUHK 14204117), the Innovation and Technology Commission (Project No. GHP-025-17SZ and GHP-028-14SZ), and the Scheme Double First Class Program, The Ministry of Education, the Ministry of Finance, and the National Development and Reform Commission.
Footnote
Conflicts of Interest: The authors have no conflicts of interest to declare.
Ethical Statement: This study was approved by the ethical review committee of the First Affiliated Hospital of Jinan University (Guangzhou, Guangdong, China), and written informed consent was obtained.
References
- Chen L, Magliano DJ, Zimmet PZ. The worldwide epidemiology of type 2 diabetes mellitus--present and future perspectives. Nat Rev Endocrinol 2011;8:228-36. [Crossref] [PubMed]
- O'Rahilly S, Barroso I, Wareham NJ. Genetic factors in type 2 diabetes: the end of the beginning? Science 2005;307:370-3. [Crossref] [PubMed]
- McCarthy MI, Froguel P. Genetic approaches to the molecular understanding of type 2 diabetes. Am J Physiol Endocrinol Metab 2002;283:E217-25. [Crossref] [PubMed]
- Huang QY, Cheng MR, Ji SL. Linkage and association studies of the susceptibility genes for type 2 diabetes. Yi Chuan Xue Bao 2006;33:573-89. [PubMed]
- Stančáková A, Laakso M. Genetics of Type 2 Diabetes. Endocr Dev 2016;31:203-20. [Crossref] [PubMed]
- Zeng C, Zhou Z, Han Y, Wen Z, Guo C, Huang S, Xiao D, Ye X, Ou M, Huang C, Ye X, Yang G, Jing C, Nie L. Interactions of TRAF6 and NLRX1 gene polymorphisms with environmental factors on the susceptibility of type 2 diabetes mellitus vascular complications in a southern Han Chinese population. J Diabetes Complications 2017;31:1652-7. [Crossref] [PubMed]
- Ling C, Cai CY, Chang BC, Shi WT, Wei FJ, Yu P, Chen LM, Li WD. MYH9 gene polymorphisms may be associated with cerebrovascular blood flow in patients with type 2 diabetes. Genet Mol Res 2015;14:1008-16. [Crossref] [PubMed]
- Cox AJ, Hugenschmidt CE, Raffield LM, Langefeld CD, Freedman BI, Williamson JD, Hsu FC, Bowden DW. Heritability and genetic association analysis of cognition in the Diabetes Heart Study. Neurobiol Aging 2014;35:1958.e3. [Crossref] [PubMed]
- Raffield LM, Cox AJ, Hugenschmidt CE, Freedman BI, Langefeld CD, Williamson JD, Hsu FC, Maldjian JA, Bowden DW. Heritability and genetic association analysis of neuroimaging measures in the Diabetes Heart Study. Neurobiol Aging 2015;36:1602.e7-15. [Crossref] [PubMed]
- Kwak SH, Park KS. Recent progress in genetic and epigenetic research on type 2 diabetes. Exp Mol Med 2016;48:e220. [Crossref] [PubMed]
- Glahn DC, Blangero J. Why endophenotype development requires families. Chin Sci Bull 2011;56:3382-4. [Crossref]
- Pearlson GD, Folley BS. Endophenotypes, dimensions, risks: is psychosis analogous to common inherited medical illnesses? Clin EEG Neurosci 2008;39:73-7. [Crossref] [PubMed]
- Glahn DC, Thompson PM, Blangero J. Neuroimaging endophenotypes: Strategies for finding genes influencing brain structure and function. Hum Brain Mapp 2007;28:488-501. [Crossref] [PubMed]
- Steward T, Pico-Perez M, Mata F, Martinez-Zalacain I, Cano M, Contreras-Rodriguez O, Fernandez-Aranda F, Yucel M, Soriano-Mas C, Verdejo-Garcia A. Emotion Regulation and Excess Weight: Impaired Affective Processing Characterized by Dysfunctional Insula Activation and Connectivity. PLoS One 2016;11:e0152150. [Crossref] [PubMed]
- van Bloemendaal L, Veltman DJ, ten Kulve JS, Drent ML, Barkhof F, Diamant M. RG IJ. Emotional eating is associated with increased brain responses to food-cues and reduced sensitivity to GLP-1 receptor activation. Obesity (Silver Spring) 2015;23:2075-82. [Crossref] [PubMed]
- Cholerton B, Baker LD, Craft S. Insulin resistance and pathological brain ageing. Diabet Med 2011;28:1463-75. [Crossref] [PubMed]
- American Diabetes Association. Standards of medical care in diabetes--2010. Diabetes Care 2010;33 Suppl 1:S11-61. [Crossref] [PubMed]
- Wahlund LO, Barkhof F, Fazekas F, Bronge L, Augustin M, Sjogren M, Wallin A, Ader H, Leys D, Pantoni L, Pasquier F, Erkinjuntti T, Scheltens P. European Task Force on Age-Related White Matter Changes. A new rating scale for age-related white matter changes applicable to MRI and CT. Stroke 2001;32:1318-22. [Crossref] [PubMed]
- Chen Z, Li L, Sun J, Ma L. Mapping the brain in type II diabetes: Voxel-based morphometry using DARTEL. Eur J Radiol 2012;81:1870-6. [Crossref] [PubMed]
- Song XW, Dong ZY, Long XY, Li SF, Zuo XN, Zhu CZ, He Y, Yan CG, Zang YF. REST: a toolkit for resting-state functional magnetic resonance imaging data processing. PLoS One 2011;6:e25031. [Crossref] [PubMed]
- Finney GR, Minagar A, Heilman KM. Assessment of Mental Status. Neurol Clin 2016;34:1-16. [Crossref] [PubMed]
- Nasreddine ZS, Phillips NA, Bedirian V, Charbonneau S, Whitehead V, Collin I, Cummings JL, Chertkow H. The Montreal Cognitive Assessment, MoCA: a brief screening tool for mild cognitive impairment. J Am Geriatr Soc 2005;53:695-9. [Crossref] [PubMed]
- O'Bryant SE, Humphreys JD, Smith GE, Ivnik RJ, Graff-Radford NR, Petersen RC, Lucas JA. Detecting dementia with the mini-mental state examination in highly educated individuals. Arch Neurol 2008;65:963-7. [PubMed]
- Samaras K, Lutgers HL, Kochan NA, Crawford JD, Campbell LV, Wen W, Slavin MJ, Baune BT, Lipnicki DM, Brodaty H, Trollor JN, Sachdev PS. The impact of glucose disorders on cognition and brain volumes in the elderly: the Sydney Memory and Ageing Study. Age (Dordr) 2014;36:977-93. [Crossref] [PubMed]
- Zhang Y, Zhang X, Zhang J, Liu C, Yuan Q, Yin X, Wei L, Cui J, Tao R, Wei P, Wang J. Gray matter volume abnormalities in type 2 diabetes mellitus with and without mild cognitive impairment. Neurosci Lett 2014;562:1-6. [Crossref] [PubMed]
- Moran C, Phan TG, Chen J, Blizzard L, Beare R, Venn A, Munch G, Wood AG, Forbes J, Greenaway TM, Pearson S, Srikanth V. Brain atrophy in type 2 diabetes: regional distribution and influence on cognition. Diabetes Care 2013;36:4036-42. [Crossref] [PubMed]
- Moulton CD, Costafreda SG, Horton P, Ismail K, Fu CHY. Meta-analyses of structural regional cerebral effects in type 1 and type 2 diabetes. Brain Imaging Behav 2015;9:651-62. [Crossref] [PubMed]
- Kumar R, Anstey KJ, Cherbuin N, Wen W, Sachdev PS. Association of type 2 diabetes with depression, brain atrophy, and reduced fine motor speed in a 60- to 64-year-old community sample. Am J Geriatr Psychiatry 2008;16:989-98. [Crossref] [PubMed]
- Wisse LE, de Bresser J, Geerlings MI, Reijmer YD, Portegies ML, Brundel M, Kappelle LJ, van der Graaf Y, Biessels GJ. Utrecht Diabetic Encephalopathy Study Group, SMART-MR Study Group. Global brain atrophy but not hippocampal atrophy is related to type 2 diabetes. J Neurol Sci 2014;344:32-6. [Crossref] [PubMed]
- García-Casares N, Berthier ML, Jorge RE, Gonzalez-Alegre P, Gutierrez Cardo A, Rioja Villodres J, Acion L, Ariza Corbo MJ, Nabrozidis A, Garcia-Arnes JA, Gonzalez-Santos P. Structural and functional brain changes in middle-aged type 2 diabetic patients: a cross-sectional study. J Alzheimers Dis 2014;40:375-86. [Crossref] [PubMed]
- García-Casares N, Garcia-Arnes JA, Rioja J, Ariza MJ, Gutierrez A, Alfaro F, Nabrozidis A, Gonzalez-Alegre P, Gonzalez-Santos P. Alzheimer's like brain changes correlate with low adiponectin plasma levels in type 2 diabetic patients. J Diabetes Complications 2016;30:281-6. [Crossref] [PubMed]
- García-Casares N, Jorge RE, Garcia-Arnes JA, Acion L, Berthier ML, Gonzalez-Alegre P, Nabrozidis A, Gutierrez A, Ariza MJ, Rioja J, Gonzalez-Santos P. Cognitive dysfunctions in middle-aged type 2 diabetic patients and neuroimaging correlations: a cross-sectional study. J Alzheimers Dis 2014;42:1337-46. [Crossref] [PubMed]
- Simmons WK, Rapuano KM, Kallman SJ, Ingeholm JE, Miller B, Gotts SJ, Avery JA, Hall KD, Martin A. Category-specific integration of homeostatic signals in caudal but not rostral human insula. Nat Neurosci 2013;16:1551-2. [Crossref] [PubMed]
- Razek AA, El-Serougy L, Abdelsalam M, Gaballa G, Talaat M. Differentiation of residual/recurrent gliomas from postradiation necrosis with arterial spin labeling and diffusion tensor magnetic resonance imaging-derived metrics. Neuroradiology 2018;60:169-77. [Crossref] [PubMed]
- Abdel Razek AAK. Arterial spin labelling and diffusion-weighted magnetic resonance imaging in differentiation of recurrent head and neck cancer from post-radiation changes. J Laryngol Otol 2018;132:923-8. [Crossref] [PubMed]
- Brundel M, Kappelle LJ, Biessels GJ. Brain imaging in type 2 diabetes. Eur Neuropsychopharmacol 2014;24:1967-81. [Crossref] [PubMed]
- Last D, Alsop DC, Abduljalil AM, Marquis RP, de Bazelaire C, Hu K, Cavallerano J, Novak V. Global and regional effects of type 2 diabetes on brain tissue volumes and cerebral vasoreactivity. Diabetes Care 2007;30:1193-9. [Crossref] [PubMed]
- Rusinek H, Ha J, Yau PL, Storey P, Tirsi A, Tsui WH, Frosch O, Azova S, Convit A. Cerebral perfusion in insulin resistance and type 2 diabetes. J Cereb Blood Flow Metab 2015;35:95-102. [Crossref] [PubMed]
- Sabri O, Hellwig D, Schreckenberger M, Schneider R, Kaiser HJ, Wagenknecht G, Mull M, Buell U. Influence of diabetes mellitus on regional cerebral glucose metabolism and regional cerebral blood flow. Nucl Med Commun 2000;21:19-29. [Crossref] [PubMed]
- El-Mewafy ZMH, Razek A, El-Eshmawy MM, El-Eneen NRA, El-Biaomy AAB. Magnetic resonance spectroscopy of the frontal region in patients with metabolic syndrome: correlation with anthropometric measurement. Pol J Radiol 2018;83:e215-9. [Crossref] [PubMed]
- Miller JL, James GA, Goldstone AP, Couch JA, He G, Driscoll DJ, Liu Y. Enhanced activation of reward mediating prefrontal regions in response to food stimuli in Prader-Willi syndrome. J Neurol Neurosurg Psychiatry 2007;78:615-9. [Crossref] [PubMed]
- Moreno-López L, Soriano-Mas C, Delgado-Rico E, Rio-Valle JS, Verdejo-Garcia A. Brain structural correlates of reward sensitivity and impulsivity in adolescents with normal and excess weight. PLoS One 2012;7:e49185. [Crossref] [PubMed]
- Beck-Nielsen H, Vaag A, Poulsen P, Gaster M. Metabolic and genetic influence on glucose metabolism in type 2 diabetic subjects--experiences from relatives and twin studies. Best Pract Res Clin Endocrinol Metab 2003;17:445-67. [Crossref] [PubMed]
- Menzies L, Achard S, Chamberlain SR, Fineberg N, Chen CH, Del Campo N, Sahakian BJ, Robbins TW, Bullmore ET. Neurocognitive endophenotypes of obsessive-compulsive disorder. Brain 2007;130:3223-36. [Crossref] [PubMed]
- Beatty J, Laughlin RE. Genomic regulation of natural variation in cortical and noncortical brain volume. BMC Neurosci 2006;7:16. [Crossref] [PubMed]