Quantitative and qualitative comparison of low- and high-cost 3D-printed heart models
Introduction
Congenital heart disease (CHD) is a type of birth defect that involves structural anomalies in the heart and major blood vessels (1). Depending on the severity of the condition, it can cause hemodynamic and functional consequences in patients, requiring corrective surgery to repair the heart (2,3). Furthermore, the forms of CHD are very diverse, including, but not limited to, double-outlet right ventricle (DORV), tetralogy of Fallot (ToF), ventricular septal defect (VSD), atrial septal defect (ASD), truncus arteriosus, single ventricle, etc. (3,4). In most cases, these conditions co-exist and vary from individual to individual, and there is thus no one-treatment-fits-all surgical option (5). It is imperative then for clinicians to achieve a comprehensive understanding of the patient’s cardiac anatomy during pre-operative assessment to prevent unexpected findings during the surgery, and subsequently reduce surgical time and mortality (6-20).
Despite this need, current visualization techniques lack the ability to provide a comprehensive viewing of the cardiac anatomy due to the medical images being interpreted from two-dimensional (2D) flat screens. Three-dimensional (3D) printing has consequently been introduced to produce models of exact replication of the heart that are both tangible and tactile (7-25). Due to the excellent geometric information that 3D-printed heart models provide, this technology has been reported to facilitate the preoperative planning of corrective surgery, improve patient-doctor communication, and enhance the learning experience of medical students (5-13,26-35). A recent multicentre study holistically explored the clinical significance of 3D-printed heart models by examining the practices of an international sample of surgeons and cardiologists from different hospitals. In 19 out of 40 cases, the 3D-printed heart models were found to be the deciding factor in altering the surgical decision (34).
Despite these advantages, the medical application of 3D printing in the domain of complex CHD is still under research and requires further validation. Several centres have adopted this technology and published case reports and series to share their experience in using 3D-printed heart models (5-12,22-24,27,33,36). The general consensus is that the cost of 3D printing remains one of the main hurdles impeding the wider application of this technology in medicine (9,19,35,37). There have been a few studies which reported on the generation of 3D-printed models using low-cost materials (6,23,24,38). However, there is no indication of whether these low-cost models are as accurate or useful as the more costly models. This study aimed to provide insights into the reduction of costs in 3D printing through the optimization of 3D printing material selection. Thus, we compared the more expensive 3D-printed model (Tango Plus) and the low-cost model [thermoplastic polyurethane (TPU)] in terms of dimensional accuracy and medical applications.
Technical note
Ten cases of de-identified cardiac computed tomography angiogram (CCTA) with CHD were retrospectively obtained from the radiology archives of two public hospitals. Of the 10 cases, 2 cases with good image quality and contrast enhancement were chosen for image segmentation. Case 1 features DORV and sub-aortic VSD, whereas case 2 demonstrates ASD. Both cases were imported to a separate workstation for segmentation using a commercially available software package, Mimics Innovation Suite software (Materialise HQ, Leuven, Belgium). Thresholding- and region-growing tools were applied to isolate the regions of interest (i.e., the blood pool) from the unwanted structures (i.e., bones, soft tissues, lungs). The mask was also manually edited if the selected region did not correctly reflect the blood pool region. The digital model of the blood pool was then exported in standard tessellation language (STL) to 3-matic, a companion software in Mimics Innovation Suite software, in order to hollow out, smoothen, and split the digital model into two compartments. An arbitrary thickness of 2 mm was also added into the blood pool surface to prevent the model from collapsing during 3D printing.
The digital models of both cases were sent for 3D printing using a low-cost material. Due to cost consideration, only the digital model of case 1 was printed using the more costly material. Tango Plus material was chosen as the “expensive” material, as it is able to reproduce models that are flexible and compressible, very much like human heart tissues. Case 1 was hence printed with a commercial Stratasys PolyJet printer (Objet Eden 260VS, Stratasys, United States), with a total printing and cleaning-up time of approximately 10 hours. The cost for 3D printing of the heart in Tango Plus was around AUD 300.
TPU 95A was chosen as the “low-cost” material. It is durable and semi-flexible, although not as flexible as Tango Plus. Both the STL files of these two cases were printed with Ultimaker 2 Extended+ 3D printer from Ultimaker BV (Geldermalsen, The Netherlands) using fused filament fabrication (FFF) technology, with an average total printing and cleaning-up time of approximately 100 hours. The average cost for 3D printing of the heart in TPU 95A was around AUD 50.
The dimensional accuracy of the 3D-printed heart models was investigated. A contrast-enhanced CT scan was performed on both the Tango Plus and TPU models of case 1 using a contrast-enhanced CT chest protocol. Both models were immersed in a water-contrast mixture of 10% contrast and 90% water to obtain a CT attenuation of 200 Hounsfield units (HU) which is similar to routine CCTA (Figure 1). Measurements were taken at 10 different anatomical locations using the “ruler” feature in the Horos software (Horos Project, licensed under the GNU Lesser General Public License, version 3.0), which is an open-source Digital Imaging and Communications in Medicine (DICOM) viewer. The results were compared with the measurements obtained from the original CCTA. In order to reduce observer bias, each measurement was repeated 3 times by 2 independent observers. It was found that the Tango Plus heart model deviated from the measurements in the original data by a 0.23 mm average, whereas the TPU model deviated from the measurements in the original data by a 0.54 mm average. However, measurements from both models were strongly correlated with those of the original CCTA (r=0.99), as demonstrated in Figures 2 and 3.
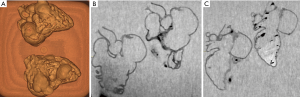
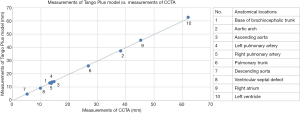
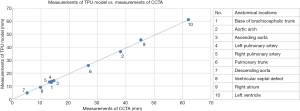
In order to compare the clinical significance of the two models, surveys were conducted involving 3 medical professionals (1 cardiac surgeon, 1 cardiologist, and 1 cardiothoracic radiologist). During the meeting with them, they were asked to qualitatively assess the original CCTA of cases 1 and 2 prior to the evaluation of the 3D-printed models, and allowed to discuss where the heart lesions were. Questionnaires were then distributed to the medical professionals to discover their opinions of both models. Each participant received 2 identical sets of questionnaires, 1 for the Tango Plus model, and 1 for the TPU model. They were requested to choose between responses of “yes”, “maybe”, and “no” with regards to the efficacy of the 3D heart models in the following areas: degree of reliability of the model, usefulness in preoperative planning, usefulness in medical education, and usefulness in communication within clinical practice. All the participants found both the models useful in the above-mentioned areas, and they found no difference between the models in terms of their medical applications. On the 3-point scale questionnaires, each respondent rated the TPU model exactly the same as the Tango Plus model. Table 1 contains the responses from each respondent with regards to the medical application of the 3D-printed heart models. It is worthwhile to note that no participant selected “no” in any of the questions, indicating that both 3D-printed models were perceived positively in terms of their efficacy.
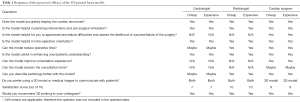
Full table
Discussion
The application of 3D printing has proliferated since its first introduction in the medical field, however mainly in the maxillofacial and orthopaedic specialties. In the past few years, 3D printing has increasingly gained attention within the cardiovascular domain, due to the potential ability of the technology to improve the patient management of cardiovascular disease (6,8,29,32). In spite of the promising results that 3D-printed heart models have shown in the current literature, the diffusion of this novel technology has been limited mainly due to its cost (9,19,35). To the best of our knowledge, there is currently no study investigating the efficiency and accuracy of the low-cost 3D printed models and whether they are comparable with the more expensive models. This preliminary study demonstrated that the low-cost models can be as useful as the expensive models in medical applications. However, its accuracy in replicating cardiac structures is less than the expensive models, and its mean difference does not fall within the mean difference reported in the other relevant articles (39). Further studies that include more cases are needed to validate this result. The low-cost model also requires a much longer duration for 3D printing—about 10 times longer than the high-cost model. Hence, it is probably not as practical when it comes to management of urgent cases.
In the free-text response questions, the participants made a few suggestions about how the 3D-printed models may be improved to bring more benefits in medical field:
“(Display of) thinner structures like valve leaflets and chordae tendineae, especially for adult valve reconstructive surgery.”
This points out one of the limitations of the 3D-printed heart models generated purely based on CT scans: very fine structures cannot be well-defined, as they are best seen on echocardiographic images. A study by Gosnell et al. integrated CT and echocardiographic scans to produce a 3D-printed heart model with an excellent replication of valve leaflets (Figure 4) (40). This method exploits the strengths of the two imaging modalities and combines them, producing a 3D-printed model that can display more anatomical and pathological information.
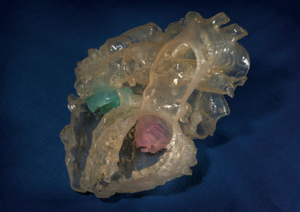
The accuracy of the 3D-printed models relies heavily on the quality of the original CT scan, especially on how well the entire blood pool is enhanced by the contrast medium. If the blood pool is not enhanced properly, manual editing is required to meticulously select the region of interest, making the process more prone to human error. One of the participants suggested it would be beneficial to develop CT imaging protocols to enhance the quality of the scans, thus reducing errors in the 3D-printed models.
It is important to bring attention to several limitations in this study. First, the study lacks generalization as there were only 2 types of 3D printing materials being investigated. There are various types of 3D printing materials in the market with different properties and costs, and their cost-effectiveness as a material for 3D-printed heart models has not yet been studied. It should not be assumed that the properties of TPU 95A and Tango Plus material can be generalized to all the other low- and high-cost 3D printed models. Second, the quantitative measurement of the 3D models’ accuracy was only carried out in one dimension: the axial plane. It is yet to be determined whether 3D printing distorts the cardiac anatomy in coronal and sagittal planes, and whether the accuracy of the 3D models is consistent in all three dimensions. This being the case, the calculated mean difference of the 3D-printed models is not completely indicative of the accuracy of the entire model. Third, detection bias may be present in the qualitative assessment due to the fact that all three participants were informed of the purpose of the study prior to the survey.
In conclusion, this technical report shows our preliminary experience in creating low-cost patient-specific 3D-printed models of CHD with similar accuracy and clinical applications as costly 3D-printed models. With further developments in 3D printing techniques and cost reductions in 3D printing materials, 3D printing will inevitably be incorporated into the diagnostic approach of daily clinical practice.
Acknowledgements
Funding: This study is supported by the National Heart Foundation of Australia (No. 101843). The authors would like to thank Mr. Werner Ducke for his help in the 3D printing of the heart model.
Footnote
Conflicts of Interest: The authors have no conflicts of interest to declare.
References
- Australian Institute of Health and Welfare [Internet]. Australian Government; c2017. How many Australians have cardiovascular disease? [cited 2018 Dec 19]. Available online: https://www.aihw.gov.au/reports-data/health-conditions-disability-deaths/heart-stroke-vascular-diseases/overview
- Yoo S, Thabit O, Kim EK, Ide H, Yim D, Dragulescu A, Seed M, Grosse-Wortmann L, van Arsdell G. 3D printing in medicine of congenital heart diseases. 3D Print Med 2015;2:1-12.
- Bruneau BG. The developmental genetics of congenital heart disease. Nature 2008;451:943-8. [Crossref] [PubMed]
- Ryan J, Plasencia J, Richardson R, Velez D, Nigro JJ, Pophal S, Frakes D. 3D printing for congenital heart disease: a single site’s initial three-year experience. 3D Print Med 2018;4:1-9.
- Bhatla P, Tretter JT, Ludomirsky A, Argilla M, Latson LA Jr, Chakravarti S, Barker PC, Yoo SJ, McElhinney DB, Wake N, Mosca RS. Utility and scope of rapid prototyping in patients with complex muscular ventricular septal defects or double-outlet right ventricle: Does it alter management decisions? Pediatr Cardiol 2017;38:103-14. [Crossref] [PubMed]
- Valverde I, Gomez G, Gonzales A, Suarez-Mejias C, Adsuar A, Coserria JF, Uribe S, Gomez-Cia T, Hosseinpour AR. Three-dimensional patient-specific cardiac model for surgical planning in Nikaidoh procedure. Cardiol Young 2015;25:698-704. [Crossref] [PubMed]
- Schmauss D, Haeberle S, Hagl C, Sodian R. Three-dimensional printing in cardiac surgery and interventional cardiology: a single-centre experience. Eur J Cardiothorac Surg 2015;47:1044-52. [Crossref] [PubMed]
- Shiraishi I, Yamagishi M, Hamaoka K, Fukuzawa M, Yagihara T. Simulative operation on congenital heart disease using rubber-like urethane stereolithographic biomodels based on 3D datasets of multislice computed tomography. Eur J Cardiothorac Surg 2010;37:302-6. [PubMed]
- Riesenkampff E, Rietdorf U, Wolf I, Schnackenburg B, Ewert P, Huebler M, Alexi-Meskishvili V, Anderson RH, Engel N, Meinzer H, Hetzer R, Berger F, Kuehne T. The practical clinical value of three-dimensional models of complex congenitally malformed hearts. J Thorac Cardiovasc Surg 2009;138:571-580. [Crossref] [PubMed]
- Mottl-Link S, Hübler M, Kühne T, Rietdorf U, Krueger JJ, Schnackenburg B, De Simone R, Berger F, Juraszek A, Meinzer H, Karck M, Hetzer R, Wolf I. Physical Models Aiding in Complex Congenital Heart Surgery. Ann Thorac Surg 2008;86:273-7. [Crossref] [PubMed]
- Ma XJ, Tao L, Chen X, Li W, Peng ZY, Chen Y, Jin J, Zhang XI, Xiong QF, Zhong ZI, Chen XF. Clinical application of three-dimensional reconstruction and rapid prototyping technology of multislice spiral computed tomography angiography for the repair of ventricular septal defect of tetralogy of Fallot. Genet Mol Res 2015;14:1301-9. [Crossref] [PubMed]
- Greil GF, Wolf I, Kuettner A, Fenchel M, Miller S, Martirosian P, Schick F, Oppitz M, Meinzer H, Sieverding I. Stereolithographic reproduction of complex cardiac morphology based on high spatial resolution imaging. Clin Res Cardiol 2007;96:176-85. [Crossref] [PubMed]
- Biglino G, Koniordou D, Gasparini M, Capelli C, Leaver L, Khambadkone S, Schievano S, Taylor AM, Wray J. Piloting the use of patient-specific cardiac models as a novel tool to facilitate communication during clinical consultations. Pediatr Cardiol 2017;38:813-8. [Crossref] [PubMed]
- Cantinotti M, Valverde I, Kutty S. Three-dimensional printed models in congenital heart disease. Int J Cardiovasc Imaging 2017;33:137-44. [Crossref] [PubMed]
- Bhatla P, Tretter JT, Chikkabyrappa S, Chakravarti S, Mosca RS. Surgical planning for a complex double-outlet right ventricle using 3D printing. Echocardiography 2017;34:802-4. [Crossref] [PubMed]
- Biglino G, Capelli C, Wray J, Schievano S, Leaver L, Khambadkone S, Giardini A, Derrick G, Jones A, Taylor AM. 3D-manufactured patient-specific models of congenital heart defects for communication in clinical practice: feasibility and acceptability. BMJ Open 2015;5:e007165. [Crossref] [PubMed]
- Biglino G, Capelli C, Leaver LK, Shievano S, Taylor AM, Wray J. Involving patients, families and medical staff in the evaluation of 3D printing models of congenital heart disease. Commun Med 2015;12:157-69. [Crossref] [PubMed]
- Biglino G, Capelli C, Koniordou D, Robertshaw D, Leaver L, Schievano S, Taylor AM, Wray J. Use of 3D models of congenital heart disease as an education tool for cardiac nurses. Congenit Heart Dis 2017;12:113-8. [Crossref] [PubMed]
- Costello JP, Olivieri L, Krieger A, Thabit O, Marshall MB, Yoo SJ, Kim PC, Jonas RA, Nath DS. Utilizing Three-Dimensional Printing Technology to Assess the Feasibility of High-Fidelity Synthetic Ventricular Septal Defect Models for Simulation in Medical Education. World J Pediatr Congenit Heart Surg 2014;5:421-6. [Crossref] [PubMed]
- Costello JP, Olivieri LJ, Su L, Krieger A, Alfares F, Thabit O, Marshall MB, Yoo SJ, Kim PC, Jonas RA, Nath DS. Incorporating three-dimensional printing into a simulation-based congenital heart disease and critical care training curriculum for resident physicians. Congenit Heart Dis 2015;10:185-90. [Crossref] [PubMed]
- Farooqi KM, Lengua CG, Weinberg AD, Nielsen JC, Sanz J. Blood Pool Segmentation Results in Superior Virtual Cardiac Models than Myocardial Segmentation for 3D Printing. Pediatr Cardiol 2016;37:1028-36. [Crossref] [PubMed]
- Farooqi KM, Gonzalez-Lengua C, Shenoy R, Sanz J, Nguyen K. Use of a three dimensional printed cardiac model to assess suitability for biventricular repair. World J Pediatr Congenit Heart Surg 2016;7:414-6. [Crossref] [PubMed]
- Garekar S, Bharati A, Chokhandre M, Mali S, Trivedi B, Changela VP, Solanki N, Gaikwad S, Agarwal V. Clinical application and multidisciplinary assessment of three dimensional printing in double outlet right ventricle with remote ventricular septal defect. World J Pediatr Congenit Heart Surg 2016;7:344-50. [Crossref] [PubMed]
- Hadeed K, Dulac Y, Acar P. Three-dimensional printing of a complex CHD to plan surgical repair. Cardiol Young 2016;26:1432-4. [Crossref] [PubMed]
- Jones TW, Seckeler MD. Use of 3D models of vascular rings and slings to improve resident education. Congenit Heart Dis 2017;12:578-82. [Crossref] [PubMed]
- Kappanayil M, Koneti NR, Kannan RR, Kottayil BP, Kumar K. Three-dimensional-printed cardiac prototypes aid surgical decision-making and preoperative planning in selected cases of complex congenital heart diseases: early experience and proof of concept in a resource-limited environment. Ann Pediatr Cardiol 2017;10:117-25. [Crossref] [PubMed]
- Kiraly L, Tofeig M, Jha NK, Talo H. Three-dimensional printed prototypes refine the anatomy of post-modified Norwood-1 complex aortic arch obstruction and allow presurgical simulation of the repair. Interact Cardiovasc Thorac Surg 2016;22:238-40. [Crossref] [PubMed]
- Loke YH, Harahsheh AS, Krieger A, Olivieri LJ. Usage of 3D models of tetralogy of Fallot for medical education: impact on learning congenital heart disease. BMC Med Educ 2017;17:54-61. [Crossref] [PubMed]
- Olejník P, Nosal M, Havran T, Furdova A, Cizmar M, Slabej M, Thurzo A, Vitovic P, Klvac M, Acel T, Masura J. Utilisation of three-dimensional printed heart models for operative planning of complex congenital heart defects. Kardiologia Polska 2017;75:495-501. [Crossref] [PubMed]
- Olivieri LJ, Krieger A, Loke YH, Nath DS, Kim PCW, Sable CA. Three-dimensional printing of intracardiac defects from three-dimensional echocardiographic images: feasibility and relative accuracy. J Am Soc Echocardiogr 2015;28:392-97. [Crossref] [PubMed]
- Olivieri LJ, Su L, Hynes CF, Krieger A, Alfares FA, Ramakrishnan K, Zurakowski D, Marshall MB, Kim PCW, Jonas RA, Nath DS. ‘‘Just-In-Time’’ simulation training using 3-D printed cardiac models after congenital cardiac surgery. World J Pediatr Congenit Heart Surg 2016;7:164-8. [Crossref] [PubMed]
- Sodian R, Weber S, Markert M, Rassoulian D, Kaczmarek I, Lueth TC, Reichart B, Daebritz S. Stereolithographic models for surgical planning in congenital heart surgery. Ann Thorac Surg 2007;83:1854-7. [Crossref] [PubMed]
- Valverde I, Gomez G, Coserria JF, Suarez-Mejias C, Uribe S, Sotelo J, Velasco MN, De Soto JS, Hossienpour A, Gomez-Cia T. 3D printed models for planning endovascular stenting in transverse aortic arch hypoplasia. Catheter Cardiovasc Interv 2015;85:1006-12. [Crossref] [PubMed]
- Valverde I, Gomez-Ciriza G, Hussain T, Suarez-Mejias C, Velasco-Forte MN, Byrne N, Ordonez A, Gonzalez-Calle A, Anderson D, Hazekamp MG, Roest AAW, Rivas-Gonzalez J, Uribe S, El-Rassi I, Simpson J, Miller O, Ruiz E, Zabala I, Mendez A, Manso B, Gallego P, Prada F, Cantinotti M, Ait-Ali L, Merino C, Parry A, Poirier N, Greil G, Razavi R, Gomez-Cia T, Hosseinpour A. Three-dimensional printed models for surgical planning of complex congenital heart defects: an international multicentre study. Eur J Cardiothorac Surg 2017;52:1139-48. [Crossref] [PubMed]
- Olivieri LJ, Zurakowski D, Ramakrishnan K, Su L, Alfares FA, Irwin MR, Heichel J, Krieger A, Nath DS. Novel, 3D Display of Heart Models in the Postoperative Care Setting Improves CICU Caregiver Confidence. World J Pediatr Congenit Heart Surg 2018;9:206-13. [Crossref] [PubMed]
- Lau IWW, Liu D, Xu L, Fan Z, Sun Z. Clinical value of patient-specific three-dimensional printing of congenital heart disease: Quantitative and qualitative assessments. Plos One 2018;13:e0194333. [Crossref] [PubMed]
- Perica E, Sun Z. Patient-specific three-dimensional printing for pre-surgical planning in hepatocellular carcinoma treatment. Quant Imaging Med Surg 2017;7:668-77. [Crossref] [PubMed]
- Liu D, Sun Z, Chaichana T, Ducke W, Fan Z. Patient-specific 3D printed models of renal tumours using home-made 3D printer in comparison with commercial 3D printer. J Med Imaging Health Inf 2018;8:303-8. [Crossref]
- Lau I, Sun Z. Three-dimensional printing in congenital heart disease: A systematic review. J Med Radiat Sci 2018;65:226-36. [Crossref] [PubMed]
- Gosnell J, Pietila T, Samuel BP, Kurup HK, Haw MP, Vettukattil JJ. Integration of Computed tomography and three-dimensional echocardiography for hybrid three-dimensional printing in congenital heart disease. J Digit Imaging 2016;29:665-9. [Crossref] [PubMed]