Quantitative assessment on coronary computed tomography angiography (CCTA) image quality: comparisons between genders and different tube voltage settings
Introduction
Multi-detector computed tomography (MDCT) created a new milestone in artifact-free cardiac and coronary artery imaging with large volume coverage (1). Advanced MDCT system (64-slice and above), widened the scope of coronary computed tomography angiography (CCTA) to visualize coronary artery wall morphology, characterize atherosclerotic plaques, identify non-stenotic plaques, and detect coronary luminal changes. Thus, the prognosis of coronary artery disease (CAD) can be provided efficiently by the less invasive CCTA method (2). Besides, Sun & Lin (3) have reported that CCTA has sensitivity and specificity of more than 97% and 87% respectively, with the use of 64-slice MDCT in the detection of CAD. Also, due to the high negative predictive value (>95%) of CCTA, it has become a reliable imaging modality for heart screening. It allows patients to choose between non invasive CCTA or an invasive coronary angiography examination for coronary artery assessment (4). These achievements and success of CCTA were attributed to rapid evolution of CT technology, which also enabled in producing CCTA with high image quality.
With the extensive use of CCTA in the current practice, radiation dose received by the patient during CCTA must not be neglected. 100 kilovoltage peak (kVp) scan protocol was proven to reduce the radiation dose to the patient significantly without compromising the image quality (5,6). However, 100 kVp scan protocol is limited to patient with low body mass index (BMI) (<25.0 kg/m2) (5,6). In the previous literature, there was an evidence of physiological differences in heart rate between genders, in which males had lower resting heart rate than females (7). Such change in resting heart rate does not affect the heart rate consistency and image quality during CCTA examination (8).
The primary objective of all imaging examinations is to produce a high diagnostic quality image. In other words, the precision and consistency of entire coronary tree to be visualized in CCTA examination is of paramount importance (9-12). When a new protocol or technique is implemented in CCTA, the image quality is an important subject matter to be discussed including the sensitivity and specificity tests to detect particular diseases and the measurements of qualitative and quantitative assessment. Several studies comparing different CT technical parameters emphasized that the image quality has to be at the optimum level for diagnosis (13-18). However, both qualitative and quantitative image quality were not discussed by some authors in terms of gender differences (16,17). Therefore, this study was carried out to quantify and compare the objective image quality between genders as well as between different tube voltages scan protocols.
Methods
Study design & population
This study was approved by the institutional ethics committee (IJNEC/05/2013) (01). Fifty five sets of CCTA were obtained retrospectively at National Heart Institute, Kuala Lumpur from January 2015 to March 2016. The images were selected randomly from patients who underwent CCTA for CAD assessment. However, patients with a history of previous heart surgery (e.g., pacemaker implantation, coronary artery bypass grafting, percutaneous coronary intervention and others) were excluded from this study.
CCTA scanning method
CCTA protocol was performed with a Somatom Definition 64-slice DSCT (Siemens Medical Solutions, Forchheim, Germany) with beam collimation of 2 mm × 32 mm × 0.6 mm, slice acquisition of 2 mm × 64 mm × 0.6 mm with the z-flying focal spot, 320 mAs per rotation and tube voltage between 100 and 120 kVp. The ECG-pulsing window was set at 30–80% of the R-R interval with a pitch of 0.2–0.43, which was automatically adapted to the heart rate.
Although the standard adult CCTA protocol is recommended with a tube voltage of 120 kVp, it is possible to reduce the tube voltage to 100 kVp in small-sized patients without compromising the image quality (19). A 100 kVp protocol was only used in patients scanned with BMI) less than 29 and 27 kg/m2 for males and females, respectively.
Contrast medium administration
A minimum of 65 mL contrast agent (Iomeron 350 mg/mL) was administered intravenously at a flow rate of 5.0–5.5 mL/s followed by 50 mL saline flush at 5 mL/s. The amount of contrast medium required for coronary CT examination was calculated according to the following formula: V = IR × ST, where V is volume in milliliters, IR is injection rate (mL/s), and ST is scanning time in seconds (20). Bolus was tracked using an automated bolus triggering technique at the ascending aorta, with a baseline threshold of 120 Hounsfield units (HU).
Quantitative image quality analysis
Quantitative image quality was measured on three types of images in Digital Imaging and Communication in Medicine (DICOM) format; consisted of reformatted axial images with a slice thickness of 0.75 mm at (I) systolic phase; (II) diastolic phase and (III) curved multiplanar reformation (cMPR) (Figure 1). Four main coronary arteries—right coronary artery (RCA), left anterior descending artery (LAD), left circumflex artery (LCx) and left main artery (LM) were evaluated individually by a single reader for all patients.
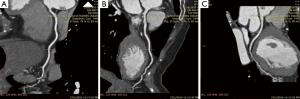
Objective image quality parameters such as signal intensity, image noise, signal-to-noise ratio (SNR) and contrast-to-noise ratio (CNR) were quantified with software Analyze version 12.0 for Windows 7 (Analyze Direct Inc., USA). Two circular regions of interest (ROIs) with the possible largest area that ranged from 3.5 to 13.8 mm2 were placed randomly along the coronary artery to obtain quantitative image quality measurements. The ROIs were placed carefully to avoid calcifications, plaques, vessels wall and any other artifacts. For quantification of signal intensity of cardiac wall, two ROIs with area ranged from 4.1 to 13.4 mm2 were placed randomly over the cardiac wall. ROIs placements were done with optimum window width/window level of 800/300.
Signal intensity was derived from mean CT attenuation values measured in HU averaged from two ROIs. Image noise was defined as the mean standard deviation of CT attenuation values within these two ROIs. SNR and CNR of coronary artery were calculated using the following formulae: SNR = SI/noise; and CNR = (SICA−SICW)/noise, where SI is signal intensity, SICA is signal intensity at coronary artery, and SICW is signal intensity at the cardiac wall.
Statistical analysis
Statistical analysis was performed using software SPSS version 22.0 for Windows (SPSS Inc., USA). All quantitative image quality parameters were presented in mean ± standard deviation. Independent t-test was performed for comparison of image quality between males and females as well as between scan protocol of 100 and 120 kVp for each coronary artery. P value <0.05 was considered statistical significant.
Results
Patient characteristics
Fifty five subjects (31 males and 24 females) with mean age of 55±10 years were involved in this study. A 100 kVp scan protocol was applied to 33 subjects (22 males and 11 females) with mean BMI value of 23.29±2.60 and 25.24±2.53 kg/m2 for males and females, respectively while 120 kVp scan protocol was applied to 22 subjects (9 males and 13 females) with mean BMI value of 31.84±4.30 and 31.67±4.49 kg/m2 for males and females, respectively.
Quantitative image quality
Six hundred and sixty coronary arteries from three types of images (220 coronary arteries from each type of images) were evaluated in this study. However, owing to an anatomical variant in one patient (LCx was absent) the images were not evaluated. Therefore, a total of 657 coronary arteries were quantified to obtain its objective image quality. All quantitative image quality parameters are presented in Table 1.
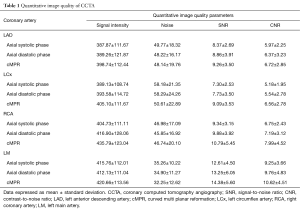
Full table
The comparison of quantitative image quality parameters between genders is presented in Table 2. There were no significant differences in all quantitative image quality parameters between males and females. However, signal intensity was significantly higher in males than females in LAD on axial images at diastolic phase (419.24±110.18 for males vs. 350.54±127.51 for females, P=0.04). Consequently, this leads to a significant increase in SNR and CNR in males as compared to females (SNR: 10.05±3.80 for males vs. 7.34±3.56 for females, P=0.01; CNR: 7.41±3.08 for males vs. 5.03±2.98 for females, P=0.01).
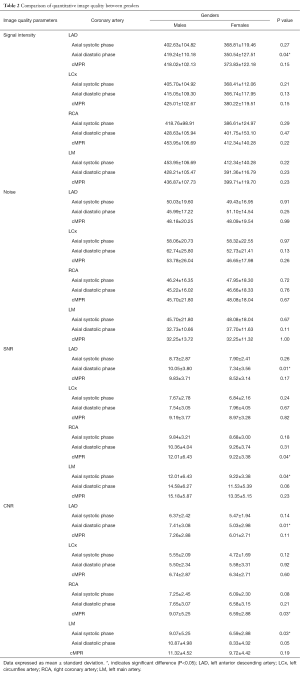
Full table
Coincidentally, both RCA on cMPR image and LM on axial images at systolic phase possessed same quantitative image quality in which there were significant differences in SNR and CNR between males and females (SNR: 12.01±6.43 for males vs. 9.22±3.38 for females, P=0.04; CNR: 9.07±5.25 for males vs. 6.59±2.88 for females, P=0.03), although no significant differences were observed for both signal intensity and noise (P=0.22 and 0.67, respectively).
On comparing quantitative image quality between 100 and 120 kVp scan protocol, all coronary arteries in all types of images showed a significantly higher signal intensity in 100 kVp scan protocol as compared to 120 kVp protocol (Figure 2, P<0.001).
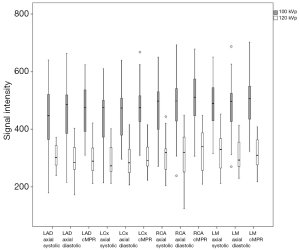
Figures 3,4 show the comparison of noise and SNR between 100 and 120 kVp scan protocol respectively. Higher noise and SNR were observed with 100 kVp scan protocol, except in LCx on the cMPR image which showed 120 kVp scan protocol produced an image with higher SNR (9.02±3.44 for 100 kVp vs. 9.20±3.75 for 120 kVp, P=0.86).
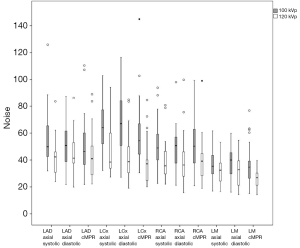
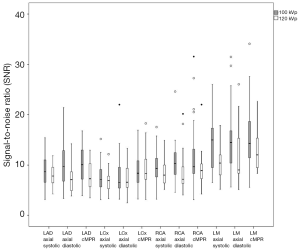
Significant difference in noise between 100 and 120 kVp scanning protocol (P<0.05), lead to non-significant difference in SNR between the two scanning protocols (P>0.05) in LAD on axial images at systolic phase, LCx on axial images at systolic and diastolic phases as well as on cMPR image, RCA on axial images at systolic phase and on cMPR image, and LM on cMPR image.
In contrast, non-significant difference in noise between 100 and 120 kVp scanning protocol (P>0.05), led to significant difference in SNR between two scanning protocols (P<0.05) in LAD on axial images at diastolic phase and on cMPR image as well as LM on axial images at systolic phase.
However, there were two coronary arteries that showed no significant differences in both noise and SNR between two scanning protocols; i.e. RCA on axial images at diastolic phase (noise: 49.25±14.17 for 100 kVp vs. 40.74±19.61 for 120 kVp, P=0.07; SNR: 10.61±3.69 for 100 kVp vs. 8.79±4.07 for 120 kVp, P=0.09) and LM on axial images at diastolic phase (noise: 36.86±10.85 for 100 kVp vs. 31.95±11.49 for 120 kVp, P=0.12; SNR: 14.58±6.19 for 100 kVp vs. 11.27±5.36 for 120 kVp, P=0.05).
Other than LCx, a significant difference in CNR between two scanning protocols was observed in all coronary arteries in all types of images (P<0.05) as presented in Figure 5. A 100 kVp scan protocol produced an image with higher CNR compared with 120 kVp scan protocol. Non-significant difference in CNR was observed in LCx on axial images at systolic phase (5.60±2.11 for 100 kVp vs. 4.53±1.51 for 120 kVp, P=0.05), axial images at diastolic phase (5.89±3.18 for 100 kVp vs. 4.98±1.96 for 120 kVp, P=0.25) and cMPR image (6.75±2.82 for 100 kVp vs. 6.27±2.77 for 120 kVp, P=0.54).
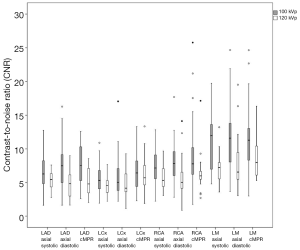
Discussion
In order to improve diagnostic accuracy of CCTA, interpreters must review three dimensional displays of CCTA interactively on 3D workstations, including trans-axial two-dimensional images (“raw data”) and post-processing images i.e., multiplanar reformation (MPR), maximum intensity projection (MIP), cMPR and volume rendering technique (VRT) reconstructions to have a better understanding of the complex coronary artery anatomy and abnormalities (21,22).
In our study, we quantified objective image quality of axial images at systolic and diastolic phase as well as cMPR images only as both axial images and cMPR images fully retained the information about the HU values. Unlike MIP technique as an example, post-processing is done based on thresholding of HU in a single voxel; this may lead to volume averaging artifacts if that particular voxel only represents part of the anatomy of interest (23). Besides, axial images with both systolic phase and diastolic phase involved as optimal reconstruction window would differ with the heart rate of the patient (24).
Quantitatively, image quality can be presented in a physical quantity such as resolution, contrast, noise and other parameters with the use of software automatically (25,26). According to Heyer et al. (27), they claimed that SNR and CNR would be the best physical parameters to judge image quality quantitatively. In spite of that, CNR is the most practicable physical parameter to depict image quality of CT system (28).
With reference to Karaca et al. (29) who classified the CNR into different image quality grade, i.e., CNR >8, high image quality; 4–8, moderate image quality; and <4, poor image quality, LAD, LCx, and RCA have moderate image quality, while LM has high image quality. This result was mainly due to the differences in relative anatomical positions of coronary arteries, in which location of LM is relatively more proximal compared to other coronary arteries. Yang et al. (30) also found out that proximal segment of coronary arteries has higher CNR over the distal segment of coronary arteries.
Moreover, ROI plays a crucial role in the quantification of objective image quality. It is an area defined by the user and is usually the first step in making any measurements. Therefore, placement of ROI is crucial in obtaining accurate measurements. Owing to the largest area of ROIs placed for quantification of objective image quality for coronary artery, the averaged CT numbers measured within that particular ROI would be more accurate compared to an ROI that only comprised of part of the anatomy of interest or CT numbers of a single pixel (31). Other than that, the ROIs used were large enough for adequate pixel sampling, and also small enough to reduce the effect of non-uniformity of CT numbers as the measurements taken were limited to coronary artery lumen while avoiding coronary artery wall (32).
Besides, the size of ROIs used for quantification of objective image quality for coronary artery and the cardiac wall is comparable. This allows fair determination of CNR as different size of ROI would affect the number of pixels included, which in turn, affects the value of CT numbers and noise measured (32).
In this study, we observed that 100 kVp scan protocol was associated with an increase in all quantitative image quality parameters comprehensively, compared to 120 kVp scan protocol. These findings correspond to work by Bischoff et al. (5), which showed an increase in signal intensity, noise, SNR, and CNR with 100 kVp scan protocol.
The increase in signal intensity could be explained by an increase in photoelectric effect, which is proportional to the atomic number (Z) and inversely proportional to the photon energy (33). With the application of low tube voltage scan protocol, the enhancement of coronary artery is increased due to the contrast medium, which contains iodine with a relatively high atomic number (Z=53), which in turn enhance the X-ray attenuation. Therefore, a reduction in tube voltage leads to an increase in attenuation by the iodinated contrast medium due to the dominance of the photoelectric event (34). As there is a better enhancement of coronary arteries by using 100 kVp scan protocol, it is possible to reduce the volume of contrast medium (34). Also, contrast medium with lower iodine content could be used as there were no differences in image quality as reported by Honoris et al. (35).
Meanwhile, the increase in image noise is mainly caused by the depletion of X-rays that reach the detectors at low tube voltage. This phenomenon occurred due to a reduction in X-ray intensity because of a decrease in tube voltage as X-ray intensity is proportional to the square of the tube voltage. Besides, the X-rays have weaker transmission strength, and there is a greater attenuation of the low energy X-ray by the filter in the CT scanner (36). This would be a major pitfall in reducing tube voltage, which can lead to grainy images that could affect diagnostic accuracy consequently.
Furthermore, an increase in signal intensity and noise resulted in an increase in SNR and CNR in our study. However, Hausleiter et al. (6) reported a reduction in SNR and CNR due to the concomitant increase in image noise with signal intensity. This is not demonstrated in our study as the increase in signal intensity outweighed the increase in image noise. Thus, 100 kVp scan protocol showed higher SNR and CNR compared to 120 kVp scan protocol.
Although we could have assumed that an individual who with BMI of 30 kg/m2 or above have excess fat mass, BMI does not distinguish between the fat and muscle compositions in the chest. Fat and muscle distributions could be different in patients with the same BMI value (37). Although genders did not show any significant changes in image quality, previous studies conducted with DSCT have shown that the effective radiation dose estimated in females is significantly higher (30% to 40%) than in male patients because of the size thickness on the chest region (38-40). However, the breast tissue is radiosensitive and keeping the radiation dose to the breast at the minimum level is of paramount importance (38).
There are some limitations in this study. ROIs were placed randomly along the coronary arteries where the best image quality is opined. This does not allow the comparison of quantitative image quality of axial images between systolic phase and diastolic phase because ROIs were not placed at the exactly same segment of the coronary artery for both phases as different coronary arteries would have its best image quality at different phases of reconstructions (24). Besides, our study did not take into consideration of other factors that may affect the image quality of CCTA, especially heart rate, and variability in heart rate.
Conclusions
This study showed that there is no significant difference in image quality of CCTA between genders. Besides, 100 kVp tube voltage scan protocol produce superior quantitative image quality of CCTA compared to 120 kVp tube voltage scan voltage; thus, 100 kVp scan protocol is highly recommended for patient whoever is compatible.
Acknowledgements
The authors would like to thank Mr. Rizal Saim from the National Heart Institute for his assistance in CT data collection.
Funding: The authors would like to thank Young Researcher Incentive Grant Scheme (GGPM-2013-099), Universiti Kebangsaan Malaysia, for financial support of the project.
Footnote
Conflicts of Interest: The authors have no conflicts of interest to declare.
Ethical Statement: This study was approved by the institutional ethics committee (IJNEC/05/2013) (01) and written informed consent was obtained from all patients.
References
- Min JK, Wann S. Indications for coronary and cardiac computed tomographic angiography. Cardiol Rev 2007;15:87-96. [Crossref] [PubMed]
- Sun Z, Choo GH, Ng KH. Coronary CT angiography: current status and continuing challenges. Br J Radiol 2012;85:495-510. [Crossref] [PubMed]
- Sun Z, Lin CH. Coronary CT angiography in coronary artery disease: from diagnosis to prevention. Heart Res Open J 2014;1:1-9. [Crossref]
- Sun Z. Multislice CT angiography in coronary artery disease: Technical developments, radiation dose and diagnostic value. World J Cardiol 2010;2:333-43. [Crossref] [PubMed]
- Bischoff B, Hein F, Meyer T, Hadamitzky M, Martinoff S, Schömig A, Hausleiter J. Impact of a reduced tube voltage on CT angiography and radiation dose: results of the PROTECTION I Study. JACC Cardiovasc Imaging 2009;2:940-6. [Crossref] [PubMed]
- Hausleiter J, Martinoff S, Hadamitzky M, Martuscelli E, Pschierer I, Feuchtner GM, Catalán-Sanz P, Czermak B, Meyer TS, Hein F, Bischoff B, Kuse M, Schömig A, Achenbach S. Image quality and radiation exposure with a low tube voltage protocol for coronary CT angiography: results of the PROTECTION II Trial. JACC Cardiovasc Imaging 2010;3:1113-23. [Crossref] [PubMed]
- Saladin KS. Anatomy & Physiology: The Unity of Form and Function, Sixth Edition. New York: McGraw-Hill Learning Solutions, 2012.
- Leschka S, Wildermuth S, Boehm T, Desbiolles L, Husmann L, Plass A, Koepfli P, Schepis T, Marincek B, Kaufmann PA, Alkadhi H. Noninvasive coronary angiography with 64-section CT: effect of average heart rate and heart rate variability on image quality. Radiology 2006;241:378-85. [Crossref] [PubMed]
- Zarb F, Rainford L, McEntee MF. Image quality assessment tools for optimization of CT images. Radiography 2010;16:147-53. [Crossref]
- Reiner BI. Hidden costs of poor image quality: a radiologist’s perspective. J Am Coll Radiol 2014;11:974-8. [Crossref] [PubMed]
- Pelgrim GJ, Oudkerk M, Vliegenthart R. Chapter 6. Computed tomography imaging of the coronary arteries. In: Baskot BG. editor. What Should We Know About Prevented, Diagnostic, and Interventional Therapy in Coronary Artery Disease. Intech, 2013.
- Ferencik M, Nomura CH, Maurovich-Horvat P, Hoffmann U, Pena AJ, Cury RC, Abbara S, Nieman K, Fatima U, Achenbach S, Brady TJ. Quantitative parameters of image quality in 64-slice computed tomography angiography of the coronary arteries. Eur J Radiol 2006;57:373-9. [Crossref] [PubMed]
- Abazid R, Smettei O, Sayed S, Harby FA, Habeeb AA, Saqqa HA, Mergania S, Selvanayagam JB. Objective and subjective image quality with prospectively gated versus ECG-controlled tube current modulation using 256-slice computed tomographic angiography. J Saudi Heart Assoc 2015;27:256-63. [Crossref] [PubMed]
- Blankstein R, Shah A, Pale R, Abbara S, Bezerra H, Bolen M, Mamuya WS, Hoffmann U, Brady TJ, Cury RC. Radiation dose and image quality of prospective triggering with dual-source cardiac computed tomography. Am J Cardiol 2009;103:1168-73. [Crossref] [PubMed]
- Hausleiter J, Meyer TS, Martuscelli E, Spagnolo P, Yamamoto H, Carrascosa P, Anger T, Lehmkuhl L, Alkadhi H, Martinoff S, Hadamitzky M, Hein F, Bischoff B, Kuse M, Schömig A, Achenbach S. Image quality and radiation exposure with prospectively ECG-triggered axial scanning for coronary CT angiography: the multicenter, multivendor, randomized PROTECTION-III Study. JACC Cardiovasc Imaging 2012;5:484-93. [Crossref] [PubMed]
- Nakashima Y, Okada M, Washida Y, Miura T, Fujimura T, Nao T, Matsunaga N. Evaluation of image quality on a per-patient, per-vessel, and per-segment basis by noninvasive coronary angiography with 64-section computed tomography: dual-source versus single-source computed tomography. Jpn J Radiol 2011;29:316-23. [Crossref] [PubMed]
- Goitein O, Beigel R, Matetzky S, Kuperstein R, Brosh S, Eshet Y, Di Segni E, Konen E. Prospectively gated coronary computed tomography angiography: uncompromised quality with markedly reduced radiation exposure in acute chest pain evaluation. Isr Med Assoc J 2011;13:463-7. [PubMed]
- Yin WH, Lu B, Li N, Han L, Hou ZH, Wu RZ, Wu YJ, Niu HX, Jiang SL, Krazinski AW, Ebersberger U, Meinel FG, Schoepf UJ. Iterative reconstruction to preserve image quality and diagnostic accuracy at reduced radiation dose in coronary CT angiography: an intraindividual comparison. JACC Cardiovasc Imaging 2013;6:1239-49. [Crossref] [PubMed]
- Alkadhi H, Stolzmann P, Scheffel H, Desbiolles L, Baumüller S, Plass A, Genoni M, Marincek B, Leschka S. Radiation dose of cardiac dual-source CT: the effect of tailoring the protocol to patient-specific parameters. Eur J Radiol 2008;68:385-91. [Crossref] [PubMed]
- Hirai N, Horiguchi J, Fujioka C, Kiguchi M, Yamamoto H, Matsuura N, Kitagawa T, Teragawa H, Kohno N, Ito K. Prospective versus retrospective ECG-gated 64-detector coronary CT angiography: assessment of image quality, stenosis, and radiation dose. Radiology 2008;248:424-30. [Crossref] [PubMed]
- Jinzaki M, Sato K, Tanami Y, Yamada M, Anzai T, Kawamura A, Ueno K, Kuribayashi S. Diagnostic accuracy of angiographic view image for the detection of coronary artery stenoses by 64-detector row CTA pilot study comparison with conventional post-processing methods and axial images alone. Circ J 2009;73:691-8. [Crossref] [PubMed]
- Raff GL, Abidov A, Achenbach S, Berman DS, Boxt LM, Budoff MJ, Cheng V, DeFrance T, Hellinger JC, Karlsberg RP, Society of Cardiovascular Computed Tomography. SCCT guidelines for the interpretation and reporting of coronary computed tomographic angiography. J Cardiovasc Comput Tomogr 2009;3:122-36. [Crossref] [PubMed]
- Catalano C, Passariello R. Multidetector-row CT angiography. Berlin: Springer Berlin Heidelberg, 2005.
- Seifarth H, Wienbeck S, Püsken M, Juergens KU, Maintz D, Vahlhaus C, Heindel W, Fischbach R. Optimal systolic and diastolic reconstruction windows for coronary CT angiography using dual-source CT. AJR Am J Roentgenol 2007;189:1317-23. [Crossref] [PubMed]
- He X, Park S. Model observers in medical imaging research. Theranostics 2013;3:774-86. [Crossref] [PubMed]
- Kamble V, Bhurchandi K. No-reference image quality assessment algorithms: A survey. Optik - International Journal for Light and Electron Optics 2015;126:1090-7.
- Heyer CM, Mohr PS, Lemburg SP, Peters SA, Nicolas V. Image quality and radiation exposure at pulmonary CT angiography with 100- or 120-kVp protocol: Prospective randomized study. Radiology 2007;245:577-83. [Crossref] [PubMed]
- Huda W, Ogden KM, Khorasani MR. Effect of dose metrics and radiation risk models when optimizing CT x-ray tube voltage. Phys Med Biol 2008;53:4719-32. [Crossref] [PubMed]
- Karaca M, Kirilmaz A, Oncel G, Oncel D, Yilmaz H, Tamci B, Gurcay B. Contrast-enhanced 64-slice computed tomography in detection and evaluation of anomalous coronary arteries. Tohoku J Exp Med 2007;213:249-59. [Crossref] [PubMed]
- Yang LH, Wu DK, Chen CY, Liu GC, Hsieh TJ, Jaw TS, Huang SY, Lin CC, Hsu JS. Quantitative assessment of image quality in 64-slice computed tomography of coronary arteries in subjects undergoing screening for coronary artery disease. Kaohsiung J Med Sci 2010;26:21-9. [Crossref] [PubMed]
- Romans LE. Computed tomography for technologists: A comprehensive text. Lippincott Williams & Wilkins, Maryland, 2010.
- Miller-Clemente R, Diaz MP, Matamoros LZ, Edyvean S. Nonlinear model of image noise: an application on computed tomography including beam hardening and image processing algorithms. Appl Math 2014;5:1240-51. [Crossref]
- Gunderson L, Tepper J. Clinical radiation oncology. 4th Edition. Philadelphia: Elsevier Inc., 2015.
- Nakayama Y, Awai K, Funama Y, Hatemura M, Imuta M, Nakaura T, Ryu D, Morishita S, Sultana S, Sato N, Yamashita Y. Abdominal CT with low tube voltage: preliminary observations about radiation dose, contrast enhancement, image quality, and noise. Radiology 2005;237:945-51. [Crossref] [PubMed]
- Honoris L, Zhong Y, Chu E, Rosenthal D, Li D, Lam F, Budoff MJ. Comparison of contrast enhancement, image quality and tolerability in coronary CT angiography using 4 contrast agents: A prospective randomized trial. Int J Cardiol 2015;186:126-8. [Crossref] [PubMed]
- Osanai M, Saito Y, Oyu K, Ishida J, Sato M, Sasaki T. Image quality and radiation dose with low tube voltage in coronary CT angiography: An experimental study with normal type and soft plaque phantom. Hirosaki Med J 2013;64:15-28.
- Wang G, Gao J, Zhao S, Sun X, Chen X, Cui X. Achieving consistent image quality and overall radiation dose reduction for coronary CT angiography with body mass index-dependent tube voltage and tube current selection. Clin Radiol 2014;69:945-51. [Crossref] [PubMed]
- Sabarudin A, Md Yusof AK, Tay MF, Ng KH, Sun Z. Dual-source CT coronary angiography: effectiveness of radiation dose reduction with lower tube voltage. Radiat Prot Dosimetry 2013;153:441-7. [Crossref] [PubMed]
- Mollet NR, Cademartiri F, van Mieghem CA, Runza G, McFadden EP, Baks T, Serruys PW, Krestin GP, de Feyter PJ. High-resolution spiral computed tomography coronary angiography in patients referred for diagnostic conventional coronary angiography. Circulation 2005;112:2318-23. [Crossref] [PubMed]
- Raff GL, Gallagher MJ, O'Neill WW, Goldstein JA. Diagnostic accuracy of noninvasive coronary angiography using 64-slice spiral computed tomography. J Am Coll Cardiol 2005;46:552-7. [Crossref] [PubMed]