Revealing the mechanism of central pain hypersensitivity in primary dysmenorrhea: evidence from neuroimaging
Introduction
Primary dysmenorrhea (PDM) is defined as menstrual pain without pelvic primary disease and is characterized by the pain in the lower abdomen radiating to the lower back or inner thighs, occasionally accompanied by dizziness, nausea, diarrhea, and other discomfort (1,2). PDM is the most common issue in menstruating women (3), with the prevalence of PDM ranging between 50% and 90% among adolescent women in studies with different populations and age groups (4,5). The exact causes of PDM are not fully understood. Related research has identified a variety of factors, including prostaglandins, anomalous uterine contractions, and psychological influences as the primary drivers of PDM (5-7). However, recent studies indicate that the brain plays a crucial role in the pathophysiology of PDM, with its involvement encompassing central sensitization, altered pain processing, regulation of neuroendocrine factors, and interactions with psychological factors (8-11).
Advancements in magnetic resonance technology, especially functional magnetic resonance imaging (fMRI), has enabled researchers to investigate the underlying central mechanism of PDM. Several studies have used fMRI to investigate the alterations of brain function and structure in patients with PDM but have yielded inconsistent results, which may be due to the variability in study groups and the different scan times in the menstrual cycle [i.e., patients with PDM being compared with healthy controls (HCs) during different time points in the menstrual cycle, lack of accounting for the interaction between the phase and group, and lack of clarity concerning the menstrual cycle].
Regardless, these studies have reported that compared with HC, patients with PDM show increased amplitude of low-frequency fluctuation (ALFF) in the medial prefrontal cortex, inferior temporal gyrus, anterior cingulate cortex, and precuneus but decreased ALFF in the thalamus, cerebellum, middle temporal gyrus, hippocampus, brainstem, postcentral gyrus, and middle frontal gyrus (12-14); increased regional homogeneity (ReHo) in the hippocampus, anterior cingulate cortex, and secondary somatosensory cortices but decreased ReHo in the cerebellum, temporal gyrus, and dorsolateral prefrontal cortex (DLPFC) (14,15); increased cerebral blood flow in the inferior frontal gyrus, precentral gyrus, posterior cingulate cortex, and superior temporal gyrus and precuneus (13,16); increased gray matter volume (GMV) in the hippocampus, anterior cingulate cortex, periaqueductal gray (PAG), hypothalamus, precuneus, and cerebellum but decreased volume in the medial prefrontal cortex, precuneus, secondary somatosensory cortices, postcentral gyrus, and superior occipital gyrus (17,18); and hypoconnectivity between the PAG and default mode network (DMN) (19).
Therefore, to comprehensively understand the mechanism of PDM, we used ALFF and ReHo to characterize the brain function change associated with PDM and voxel-based morphometry (VBM) to assess the brain structure associated with PDM. Furthermore, as the PAG is the key region of the pain modulation system and may serve a crucial role in the pathophysiology of PDM (17,19-22), we used the PAG as a region of interest to conduct seed-based whole-brain functional connectivity (FC) analysis. The aim of this study was to (I) clarify the long-term effects of PDM on the brain by comparing the patients with PDM during the nonpain phase (PDM-NP) with HCs and (II) to identify the mechanism of central pain hypersensitivity in individuals with PDM experiencing menstrual pain by comparing the patients with PDM during the pain phase (PDM-P) with those during the PDM-NP. We present this article in accordance with the STROBE reporting checklist (available at https://qims.amegroups.com/article/view/10.21037/qims-23-1687/rc).
Methods
Participants
This study employed a cross-sectional design with prospective data collection. Patients with PDM who had undergone two fMRI scans during the PDM-P and the PDM-NP were recruited in this study. The patients with PDM were screened by a gynecologist with the help of a sonographer conducting pelvic ultrasound to detect pelvic organ disease. The inclusion criteria for patients were as follows: (I) diagnosed as PDM according to the Primary Dysmenorrhea Consensus Guideline (6), (II) visual analogue scale (VAS) ≥4 in the past 6 months, (III) in the pain-free phase of the menstrual cycle, (IV) nulliparous status, and (V) aged 18–35 years with right-handedness. Meanwhile, the exclusion criteria were the following: (I) contraindications to MRI scanning; (II) participation in other clinical trials during the same period, (III) intake of analgesics or sedatives within 1 week before MRI examination; and (IV) combined with relevant medical history and other examinations, accompaniment of life-threatening primary diseases, mental disorders, or other suspected diseases that could not be excluded. The demographic and clinical characteristics, including age, years of education, smoking status, drinking status, past medical history, body mass index (BMI), visual analog scale (VAS), Cox Menstruation Symptom Scale-severity subscale (CMSS-s), self-rating depression scale (SDS), and self-rating anxiety scale (SAS) were all recorded. In the nonpain phase, patients with PDM were asked to record the average VAS and CMSS-s scores for the previous 6 months. In the pain phase, patients with PDM were asked to record the current VAS and CMSS-s scores. HCs without a history of PDM were also recruited in this study.
The study was carried out in accordance with the Declaration of Helsinki (as revised in 2013) and was approved by the Ethics Committee of Hangzhou TCM Hospital Affiliated to Zhejiang Chinese Medical University (No. 2022ky079). Written informed consent was obtained from each participant.
MRI acquisition
All scans were conducted on the 3.0 Tesla MR scanner (Discovery MR750 scanner, GE HealthCare) equipped with a 32-channel head coil. All participants were wearing earplugs and were instructed to lie on the scanning bed supine, with their head in the head coil. Cushioned pads were used to fix the head to reduce the motion artifact. All participants were asked to keep their eyes closed and to think of nothing but to stay awake during the scan. First, the conventional MRI session including T1-weighted imaging (T1WI), T2-weighted image, T2- and fluid-attenuated inversion recovery (T2-FLAIR), and diffusion-weighted imaging (DWI) to ensure that participants had no intracranial lesions. High-resolution T1W structural images were acquired with the Sagittal three-dimensional (3D) T1-weighted brain volume imaging (BRAVO) sequence [repetition time (TR) =8.2 ms, echo time (TE) =3.2 ms, flip angle =12, matrix =256×256, slice thickness =1.2 mm with no gaps]. Functional images were obtained with the gradient-echo echo-planar imaging (EPI) sequence [TR =2,000 ms, TE =35 ms, matrix =64×64, field of view (FOV)=240×240 mm, FA =90◦, slice thickness =5 mm with no gaps, number of slices =30].
Data processing
A similar data processing approach was used as in our previous studies (23,24), with functional and structural images being preprocessed using the Data Processing & Analysis of Brain Imaging (DPABI) tool (http://rfmri.org/dpabi) (25). The steps were as follows: (I) conversion of Digital Imaging and Communications in Medicine (DICOM) format to Neuroimaging Informatics Technology Initiative (NIfTI) format; (II) removal of the first 10 time points; (III) slice timing correction; (IV) segmentation; (V) head motion correction; (VI) normalization; (VII) calculation of ALFF; (VIII) filtering; (IX) calculation of ReHo ; (X) seed-based FC analysis, in which seeds were defined as bilateral ventrolateral PAG (±4, –26, –14) based on a previous study (26); and (XI) smoothing. For details, see Figure 1.
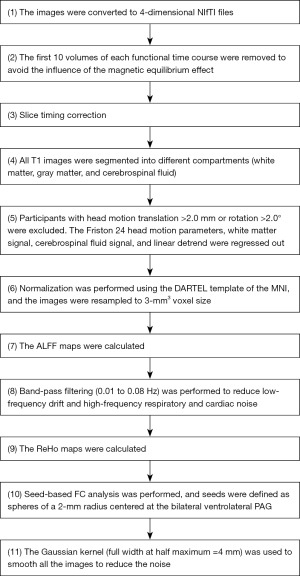
Statistical analysis
For demographic and clinical characteristics data, statistical analyses were performed using SPSS software 25.0 (IMB Corp.). For the data conforming to normal distribution, paired t-tests were used for intragroup comparisons, while two-sample t-tests were used for intergroup comparisons. Pearson correlation coefficient was used to evaluate the associations between the abnormal brain regions and the clinical information of the patients. Meanwhile, the rank-sum test and Spearman rank correlation coefficient were used for nonnormally distributed data. The significance level was set at a threshold of a two-tailed P value <0.05.
For fMRI data, statistical analyses were performed using DPABI software. Two-sample t-tests were performed to determine the differences between patients in the PDM-NP and HCs. A paired t-test was performed to explore the differences between patients in the PDM-P and those in the PDM-NP. Multiple comparison correction was performed using the Gaussian random field (GRF) with associated Bonferroni correction being performed with a voxel-wise P value <0.0005 and a cluster-wise P value <0.05, which is a strict threshold and has high test-retest reliability and replicability (27). Pearson correlation analyses were employed to evaluate the associations between the z-scores of the cluster and the clinical information of the patients.
Results
Demographic and clinical characteristics
Initially, 40 patients with PDM and 20 HCs were recruited in the study, with complete fMRI scan during the nonpain phase. Thirteen patients dropped out due to scheduling conflicts and severe pain during the pain phase, in that they needed to rest at home and were unable to come to the hospital to complete the fMRI scan. Therefore, 27 patients with PDM underwent the fMRI scans during the pain phase. Additionally, two patients with PDM during the pain phase were excluded due to excessive head motion. Finally, 25 patients with PDM and 20 HCs were included in the final analyses (Figure 2).
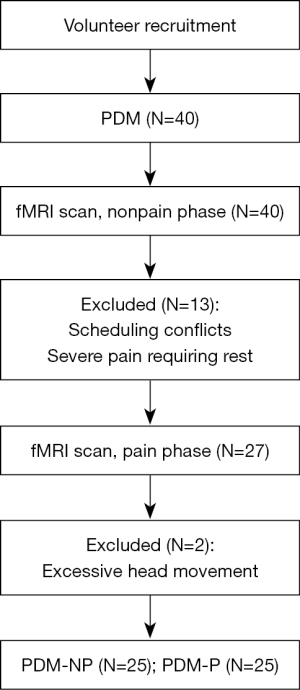
The demographic and clinical characteristics of the patients with PDM and HCs are shown in Table 1. None of the patients with PDM or HCs engaged in smoking or the drinking of alcohol. The menstrual phase of patients with PDM was longer than that of the HCs (P=0.03; Table 1). There were no statistically significant differences in the age, education, BMI, menstrual cycle, onset age of menophania, SAS, or SDS scores between patients with PDMs and HCs (P>0.05; Table 1).
Table 1
Characteristics | PDM (n=25) | HC (n=20) | P value | Cohen’s d |
---|---|---|---|---|
Age (years) | 24.40±2.06 | 25.30±2.22 | 0.16 | −0.421 |
Education (years) | 17.96±1.56 | 18.25±1.51 | 0.53 | −0.188 |
Body mass index (kg/m2) | 19.93±1.71 | 20.04±1.94 | 0.84 | −0.061 |
Onset age of menophania (years) | 13.28±1.02 | 13.30±1.26 | 0.95 | −0.018 |
Menstrual cycle (days) | 32.52±4.81 | 31.65±4.59 | 0.54 | 0.184 |
Menstrual phase (days) | 6.56±0.96 | 5.85±1.22 | 0.03 | 0.654 |
History of PDM (years) | 6.92±3.16 | – | – | – |
SAS scores | 32.00±7.03 | 28.50±4.00 | 0.05 | 0.594 |
SDS scores | 31.84±7.58 | 29.15±7.16 | 0.23 | 0.363 |
PDM, primary dysmenorrhea; HC, healthy control; SD, standard deviation; SAS, self-rating anxiety scale; SDS, self-rating depression scale.
The differences in clinical characteristics between patients in the PDM-P and those in the PDM-NP are shown in Table 2. The SDS score during pain phase was higher than that in the nonpain phase (P=0.04; Table 2); moreover, the VAS score during pain phase was lower than the average score reported in the previous 6 months (P=0.04; Table 2).
Table 2
Characteristics | Pain (n=25) | Nonpain (n=25) | P value | Cohen’s d |
---|---|---|---|---|
SAS scores | 33.64±8.92 | 32.00±7.03 | 0.15 | 0.291 |
SDS scores | 34.64±9.42 | 31.84±7.58 | 0.04 | 0.414 |
VAS scores | 4.96±1.09 | 5.68±1.60 | 0.04 | −0.425 |
CMSS-s scores | 13.56±7.98 | 14.40±7.31 | 0.45 | −0.153 |
PDM, primary dysmenorrhea; SD, standard deviation; SAS, self-rating anxiety scale; SDS, self-rating depression scale; VAS, visual analog scale; CMSS-s, Cox Menstruation Symptom Scale-severity subscale.
GMV, ALFF, ReHo, and FC results
There were no statistically significant differences between patients in the PDM-NP and HCs. Compared with patients in the PDM-NP, those in the PDM-P showed increased ReHo in the left DLPFC (Table 3 and Figure 3) but decreased FC between the PAG and several regions, including the right superior parietal gyrus, bilateral inferior parietal gyrus, right calcarine gyrus, left superior occipital gyrus, left precentral gyrus, right DLPFC. and left crus I of cerebellar hemisphere (Table 3 and Figure 4). In addition, the FC between left PAG and left crus I of the cerebellar hemisphere was negatively correlated with VAS score during the pain phase (r=−0.478; P=0.016) (Figure 5).
Table 3
Contrast | Voxels | Brain region | MNI coordinate | t value | ||
---|---|---|---|---|---|---|
X | Y | Z | ||||
ReHo | ||||||
Pain > nonpain | 11 | L dorsolateral prefrontal cortex | −12 | 51 | 42 | 6.2622 |
Fc_l_pag | ||||||
Pain < nonpain | 34 | R superior parietal gyrus | 21 | −57 | 51 | −4.7103 |
Pain < nonpain | 28 | L inferior parietal gyrus | −24 | −54 | 51 | −5.4097 |
Pain < nonpain | 28 | R calcarine gyrus | 27 | −72 | 12 | −5.5317 |
Pain < nonpain | 42 | L superior occipital gyrus | −15 | −84 | 12 | −5.4554 |
Pain < nonpain | 40 | L crus I of cerebellar hemisphere | −30 | −87 | −21 | −5.2779 |
Fc_r_pag | ||||||
Pain < nonpain | 20 | L precentral gyrus | −42 | −3 | 54 | −5.7316 |
Pain < nonpain | 31 | R inferior parietal gyrus | 42 | −39 | 45 | −5.3688 |
Pain < nonpain | 69 | R dorsolateral prefrontal cortex | 42 | 51 | −6 | −5.7665 |
Pain < nonpain | 21 | L crus I of cerebellar hemisphere | −30 | −84 | −21 | −5.3087 |
ReHo, regional homogeneity; FC, functional connectivity; PDM, primary dysmenorrhea; MNI, Montreal Neurological Institute; L, left; R, right; Fc_l_pag, brain regions with altered functional connectivity to the left periaqueductal gray; Fc_r_pag, brain regions with altered functional connectivity to the right periaqueductal gray.
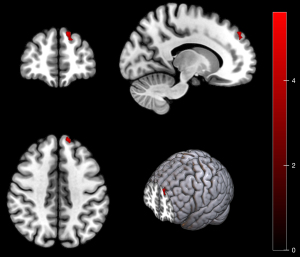
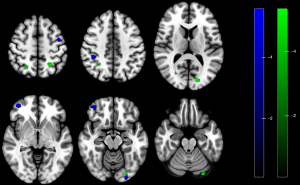
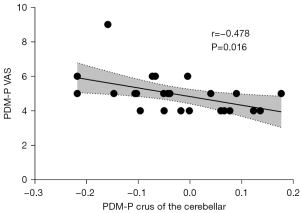
Discussion
This study employed ReHo, ALFF, FC. and VBM to explore the mechanism of central pain hypersensitivity of PDM during menstrual pain by comparing patients in the PDM-P with those in the PDM-NP. In addition, the long-term effects of PDM were investigated by comparing patients in the PDM-NP with HCs. The findings revealed that there were no functional or structural differences between patients in the PDM-NP and HCs. However, there was evidence of altered brain function and FC patterns of the PAG during the pain phase.
Patients in the PDM-P showed increased ReHo in the DLPFC compared with those in the PDM-NP. The ReHo measures the similarity of the time series of a given voxel to those of its nearest neighbors and reflects the coherence of spontaneous neuronal activity (28). The DLPFC is an important region in the pain modulatory system, is involved in cognitive control over pain, and acts as an interface between cognitive processing and pain regulation (29). Stimulation of the DLPFC can exert an immediate analgesic effect and reduce the unpleasantness of pain (30,31). A previous study demonstrated that stimulation of the DLPFC can improve anxiety and functionality in patients with PDM (32). Therefore, our study showed that increased coherence of spontaneous neuronal activity in the DLPFC may reflect defense or self-protection during the pain phase. However, the PDM-P also showed decreased FC between the PAG and DLPFC. The PAG and DLPFC are considered to be important regions in the descending pain modulation system, and the DLPFC might play a role in pain suppression by regulating the subcortical pathway (30,33,34). Therefore, the disorder of descending pain the modulation system may contribute to the mechanisms of the central pain hypersensitivity of PDM.
This study found there to be decreased FC between the PAG and the inferior parietal gyrus and crus I of cerebellar hemisphere in patients in the PDM-P compared with those in the PDM-NP. These regions are considered to be the components of the DMN (35,36). The DMN is active in the resting state and has been implicated in the self-referential judgments, probing social cognition and communication, episodic memory operation, language comprehension, and semantic processing (37). It also participates in the pain process, and alterations in DMN function and the disrupted communication between the PAG and DMN have been reported in multiple pain conditions (38-41). Research also indicates there to be abnormal brain function and structural changes in the DMN in patients with PDM, and the maladaptive hypoconnectivity between the PAG and DMN has been identified as the central susceptibility to subsequent development of various functional disorders later in life in patients with PDM (12,15,17,19,42). Furthermore, our results showed that the FC between the PAG and cerebellum was negatively correlated with VAS in the PDM-P group. This suggests that the lower the FC between these two regions is, the more severe the symptoms of PDM. Therefore, our results provide evidence suggesting that the hypoconnectivity between the PAG and DMN may be one of the mechanisms of central pain hypersensitivity of PDM.
The superior parietal gyrus is part of the secondary sensory cortex, which is associated with the high-level integration function and is linked to the feeling of pain and painful emotions (43-46). It may be involved in analgesic effects, and its stimulation may lead to impaired judgment of pain intensity and reduce perceived pain intensity (47). The altered function and structure of the superior parietal gyrus has also been found in other pain disorders (48-50). Therefore, the hypoconnectivity between the PAG and the superior parietal gyrus in this study may suggest the disorder of the descending pain modulation system.
The calcarine gyrus and occipital gyrus are widely known as being associated with visual information processing. However, the occipital lobe has also been found to be involved in pain processing, the altered function and structure of which have been reported in other pain conditions (23,51). One study reported reduced thickness in the superior occipital gyrus in patients with PDM compared with HCs (18). There may be an integration of higher regions of the visual system and pain structure in pain processing (18). Therefore, the altered FC between the PAG and occipital lobe in patients with PDM needs to be further explored.
This study has some limitations which should be mentioned. First, the VAS score during the pain phase was lower than the average for the previous 6 months, which might have resulted from the patients with severe pain being excluded due to the inability to complete the fMRI scan. The potential effects of the different severity of the pain on the results cannot be ignored. Second, the SDS score during pain phase was higher than that in the nonpain phase. Thus, the possibility that emotions affected the results cannot be ruled out. Third, the family history of dysmenorrhea of patients with PDM was not recorded. The potential effects of this risk factor on the results should be noted. Fourth, we did not record laboratory indicators such as estrogen and progesterone. Future experiments on this subject should examine these factors.
Conclusions
The present study revealed that long-term PDM was not associated with functional or structural changes in the brain. However, the function of brain was changed during the pain phase, suggesting an important role for the brain in the pathophysiology of PDM. The mechanisms of central pain hypersensitivity of PDM may be related to the disorder of the FC between the PAG and various brain regions involved in descending pain modulation system, the DMN, and the occipital lobe. These findings could help us better understand the pathophysiology of PDM from a neuroimaging perspective, opening a new frontier in developing novel therapies for PDM treatment.
Acknowledgments
Funding: This project was supported by
Footnote
Reporting Checklist: The authors have completed the STROBE reporting checklist. Available at https://qims.amegroups.com/article/view/10.21037/qims-23-1687/rc
Conflicts of Interest: All authors have completed the ICMJE uniform disclosure form (available at https://qims.amegroups.com/article/view/10.21037/qims-23-1687/coif). The authors have no conflicts of interest to declare.
Ethical Statement: The authors are accountable for all aspects of the work in ensuring that questions related to the accuracy or integrity of any part of the work are appropriately investigated and resolved. The study was carried out in accordance with the Declaration of Helsinki (as revised in 2013) and was approved by the Ethics Committee of Hangzhou TCM Hospital Affiliated to Zhejiang Chinese Medical University (No. 2022ky079). Written informed consent was obtained from each participant.
Open Access Statement: This is an Open Access article distributed in accordance with the Creative Commons Attribution-NonCommercial-NoDerivs 4.0 International License (CC BY-NC-ND 4.0), which permits the non-commercial replication and distribution of the article with the strict proviso that no changes or edits are made and the original work is properly cited (including links to both the formal publication through the relevant DOI and the license). See: https://creativecommons.org/licenses/by-nc-nd/4.0/.
References
- Kho KA, Shields JK. Diagnosis and Management of Primary Dysmenorrhea. JAMA 2020;323:268-9. [Crossref] [PubMed]
- Ferries-Rowe E, Corey E, Archer JS. Primary Dysmenorrhea: Diagnosis and Therapy. Obstet Gynecol 2020;136:1047-58. [Crossref] [PubMed]
- Sharghi M, Mansurkhani SM, Larky DA, Kooti W, Niksefat M, Firoozbakht M, Behzadifar M, Azami M, Servatyari K, Jouybari L. An update and systematic review on the treatment of primary dysmenorrhea. JBRA Assist Reprod 2019;23:51-7. [Crossref] [PubMed]
- Ziaei S, Zakeri M, Kazemnejad A. A randomised controlled trial of vitamin E in the treatment of primary dysmenorrhoea. BJOG 2005;112:466-9. [Crossref] [PubMed]
- ACOG Committee Opinion No. 760 Summary: Dysmenorrhea and Endometriosis in the Adolescent. Obstet Gynecol 2018;132:1517-8. [Crossref] [PubMed]
- Burnett M, Lemyre M. No. 345-Primary Dysmenorrhea Consensus Guideline. J Obstet Gynaecol Can 2017;39:585-95. [Crossref] [PubMed]
- Itani R, Soubra L, Karout S, Rahme D, Karout L, Khojah HMJ. Primary Dysmenorrhea: Pathophysiology, Diagnosis, and Treatment Updates. Korean J Fam Med 2022;43:101-8. [Crossref] [PubMed]
- Clemenza S, Vannuccini S, Capezzuoli T, Meleca CI, Pampaloni F, Petraglia F. Is primary dysmenorrhea a precursor of future endometriosis development? Gynecol Endocrinol 2021;37:287-93. [Crossref] [PubMed]
- Lee LC, Chen YY, Li WC, Yang CJ, Liu CH, Low I, Chao HT, Chen LF, Hsieh JC. Adaptive neuroplasticity in the default mode network contributing to absence of central sensitization in primary dysmenorrhea. Front Neurosci 2023;17:1094988. [Crossref] [PubMed]
- Iacovides S, Avidon I, Baker FC. What we know about primary dysmenorrhea today: a critical review. Hum Reprod Update 2015;21:762-78. [Crossref] [PubMed]
- De Sanctis V, Soliman A, Bernasconi S, Bianchin L, Bona G, Bozzola M, Buzi F, De Sanctis C, Tonini G, Rigon F, Perissinotto E. Primary Dysmenorrhea in Adolescents: Prevalence, Impact and Recent Knowledge. Pediatr Endocrinol Rev 2015;13:512-20.
- Liu P, Liu Y, Wang G, Yang X, Jin L, Sun J, Qin W. Aberrant default mode network in patients with primary dysmenorrhea: a fMRI study. Brain Imaging Behav 2017;11:1479-85. [Crossref] [PubMed]
- Zhang YN, Huo JW, Huang YR, Hao Y, Chen ZY. Altered amplitude of low-frequency fluctuation and regional cerebral blood flow in females with primary dysmenorrhea: a resting-state fMRI and arterial spin labeling study. J Pain Res 2019;12:1243-50. [Crossref] [PubMed]
- Liu N, Li Y, Hong Y, Huo J, Chang T, Wang H, Huang Y, Li W, Zhang Y. Altered brain activities in mesocorticolimbic pathway in primary dysmenorrhea patients of long-term menstrual pain. Front Neurosci 2023;17:1098573. [Crossref] [PubMed]
- Jin L, Yang X, Liu P, Sun J, Chen F, Xu Z, Qin W, Tian J. Dynamic abnormalities of spontaneous brain activity in women with primary dysmenorrhea. J Pain Res 2017;10:699-707. [Crossref] [PubMed]
- Zhang YN, Huang YR, Liu JL, Zhang FQ, Zhang BY, Wu JC, Ma Y, Xia J, Hao Y, Huo JW. Aberrant resting-state cerebral blood flow and its connectivity in primary dysmenorrhea on arterial spin labeling MRI. Magn Reson Imaging 2020;73:84-90. [Crossref] [PubMed]
- Tu CH, Niddam DM, Chao HT, Chen LF, Chen YS, Wu YT, Yeh TC, Lirng JF, Hsieh JC. Brain morphological changes associated with cyclic menstrual pain. Pain 2010;150:462-8. [Crossref] [PubMed]
- Liu N, Huo J, Li Y, Hao Y, Dai N, Wu J, Liu Z, Zhang Y, Huang Y. Changes in brain structure and related functional connectivity during menstruation in women with primary dysmenorrhea. Quant Imaging Med Surg 2023;13:1071-82. [Crossref] [PubMed]
- Wei SY, Chao HT, Tu CH, Li WC, Low I, Chuang CY, Chen LF, Hsieh JC. Changes in functional connectivity of pain modulatory systems in women with primary dysmenorrhea. Pain 2016;157:92-102. [Crossref] [PubMed]
- Tu CH, Lee YC, Chen YY, Chen CM, Lu WC, Chen YH, Yang ST. Acupuncture Treatment Associated with Functional Connectivity Changes in Primary Dysmenorrhea: A Resting State fMRI Study. J Clin Med 2021;10:4731. [Crossref] [PubMed]
- Benarroch EE. Descending monoaminergic pain modulation: bidirectional control and clinical relevance. Neurology 2008;71:217-21. [Crossref] [PubMed]
- Eippert F, Bingel U, Schoell ED, Yacubian J, Klinger R, Lorenz J, Büchel C. Activation of the opioidergic descending pain control system underlies placebo analgesia. Neuron 2009;63:533-43. [Crossref] [PubMed]
- Zhang L, Yu W, Zhang Z, Xu M, Cui F, Song W, Cao Z. Altered brain activity and functional connectivity in migraine without aura during and outside attack. Neurol Res 2023;45:603-9. [Crossref] [PubMed]
- Jin P, Cui F, Xu M, Ren Y, Zhang L. Altered brain function and structure pre- and post- COVID-19 infection: a longitudinal study. Neurol Sci 2024;45:1-9. [Crossref] [PubMed]
- Yan CG, Wang XD, Zuo XN, Zang YF. DPABI: Data Processing & Analysis for (Resting-State) Brain Imaging. Neuroinformatics 2016;14:339-51. [Crossref] [PubMed]
- Chen Z, Chen X, Liu M, Liu S, Ma L, Yu S. Disrupted functional connectivity of periaqueductal gray subregions in episodic migraine. J Headache Pain 2017;18:36. [Crossref] [PubMed]
- Chen X, Lu B, Yan CG. Reproducibility of R-fMRI metrics on the impact of different strategies for multiple comparison correction and sample sizes. Hum Brain Mapp 2018;39:300-18. [Crossref] [PubMed]
- Zang Y, Jiang T, Lu Y, He Y, Tian L. Regional homogeneity approach to fMRI data analysis. Neuroimage 2004;22:394-400. [Crossref] [PubMed]
- Seminowicz DA, Moayedi M. The Dorsolateral Prefrontal Cortex in Acute and Chronic Pain. J Pain 2017;18:1027-35. [Crossref] [PubMed]
- Lorenz J, Minoshima S, Casey KL. Keeping pain out of mind: the role of the dorsolateral prefrontal cortex in pain modulation. Brain 2003;126:1079-91. [Crossref] [PubMed]
- Li X, Yao J, Lin X, Chen S, Jin R, Peng W. Transcranial random noise stimulation over the left dorsolateral prefrontal cortex attenuates pain expectation and perception. Clin Neurophysiol 2023;147:1-10. [Crossref] [PubMed]
- Dutra LRDV, Pegado R, Silva LK, da Silva Dantas H, Câmara HA, Silva-Filho EM, Correia GN, Micussi MTABC. Modulating Anxiety and Functional Capacity with Anodal tDCS Over the Left Dorsolateral Prefrontal Cortex in Primary Dysmenorrhea. Int J Womens Health 2020;12:243-51. [Crossref] [PubMed]
- Akerman S, Holland PR, Goadsby PJ. Diencephalic and brainstem mechanisms in migraine. Nat Rev Neurosci 2011;12:570-84. [Crossref] [PubMed]
- Tracey I. Neuroimaging of pain mechanisms. Curr Opin Support Palliat Care 2007;1:109-16. [Crossref] [PubMed]
- Buckner RL, Andrews-Hanna JR, Schacter DL. The brain's default network: anatomy, function, and relevance to disease. Ann N Y Acad Sci 2008;1124:1-38. [Crossref] [PubMed]
- Andrews-Hanna JR, Smallwood J, Spreng RN. The default network and self-generated thought: component processes, dynamic control, and clinical relevance. Ann N Y Acad Sci 2014;1316:29-52. [Crossref] [PubMed]
- Menon V. 20 years of the default mode network: A review and synthesis. Neuron 2023;111:2469-87. [Crossref] [PubMed]
- Alhajri N, Boudreau SA, Mouraux A, Graven-Nielsen T. Pain-free default mode network connectivity contributes to tonic experimental pain intensity beyond the role of negative mood and other pain-related factors. Eur J Pain 2023;27:995-1005. [Crossref] [PubMed]
- Alshelh Z, Marciszewski KK, Akhter R, Di Pietro F, Mills EP, Vickers ER, Peck CC, Murray GM, Henderson LA. Disruption of default mode network dynamics in acute and chronic pain states. Neuroimage Clin 2018;17:222-31. [Crossref] [PubMed]
- Zhang YN, Xing XX, Chen L, Dong X, Pan HT, Hua XY, Wang K. Modification of the resting-state network involved at different stages of neuropathic pain. Neurosci Lett 2022;789:136866. [Crossref] [PubMed]
- Chen F, Zhang S, Li P, Xu K, Liu C, Geng B, Piao R, Liu P. Disruption of Periaqueductal Gray-default Mode Network Functional Connectivity in Patients with Crohn's Disease with Abdominal Pain. Neuroscience 2023;517:96-104. [Crossref] [PubMed]
- Wu TH, Tu CH, Chao HT, Li WC, Low I, Chuang CY, Yeh TC, Cheng CM, Chou CC, Chen LF, Hsieh JC. Dynamic Changes of Functional Pain Connectome in Women with Primary Dysmenorrhea. Sci Rep 2016;6:24543. [Crossref] [PubMed]
- Ma J, Wu JJ, Hua XY, Zheng MX, Huo BB, Xing XX, Feng SY, Li B, Xu J. Alterations in brain structure and function in patients with osteonecrosis of the femoral head: a multimodal MRI study. PeerJ 2021;9:e11759. [Crossref] [PubMed]
- Li JL, Yan CQ, Wang X, Zhang S, Zhang N, Hu SQ, Wang LQ, Liu CZ. Brain Functional Alternations of the Pain-related Emotional and Cognitive Regions in Patients with Chronic Shoulder Pain. J Pain Res 2020;13:575-83. [Crossref] [PubMed]
- May A. Structural brain imaging: a window into chronic pain. Neuroscientist 2011;17:209-20. [Crossref] [PubMed]
- Derbyshire SW. A systematic review of neuroimaging data during visceral stimulation. Am J Gastroenterol 2003;98:12-20. [Crossref] [PubMed]
- Valmunen T, Pertovaara A, Taiminen T, Virtanen A, Parkkola R, Jääskeläinen SK. Modulation of facial sensitivity by navigated rTMS in healthy subjects. Pain 2009;142:149-58. [Crossref] [PubMed]
- Wang ZW, Yin ZH, Wang X, Zhang YT, Xu T, Du JR, Wen Y, Liao HQ, Zhao Y, Liang FR, Zhao L. Brain structural and functional changes during menstrual migraine: Relationships with pain. Front Mol Neurosci 2022;15:967103. [Crossref] [PubMed]
- Zhang J, Wu YL, Su J, Yao Q, Wang M, Li GF, Zhao R, Shi YH, Zhao Y, Zhang Q, Lu H, Xu S, Qin Z, Cui GH, Li J, Liu JR, Du X. Assessment of gray and white matter structural alterations in migraineurs without aura. J Headache Pain 2017;18:74. [Crossref] [PubMed]
- Bu C, Ren H, Lv Q, Bu H, Gao X, Zheng R, Huang H, Wang W, Wei Y, Cheng J, Zhang Y. Alteration of static and dynamic intrinsic brain activity induced by short-term spinal cord stimulation in postherpetic neuralgia patients. Front Neurosci 2023;17:1254514. [Crossref] [PubMed]
- Soheili-Nezhad S, Sedghi A, Schweser F, Eslami Shahr Babaki A, Jahanshad N, Thompson PM, Beckmann CF, Sprooten E, Toghae M. Structural and Functional Reorganization of the Brain in Migraine Without Aura. Front Neurol 2019;10:442. [Crossref] [PubMed]