Evaluating left atrial appendage function in a subtype of non-valvular atrial fibrillation using transesophageal echocardiography combined with two-dimensional speckle tracking
Introduction
Atrial fibrillation (AF) is the most common type of arrhythmia worldwide and is a major risk factor for cardioembolic stroke (1). Thrombosis is usually found in the left atrial appendage (LAA) in patients with AF, due to reduced LAA contractility and blood stasis (2). Therefore, for patients with AF, attention should be paid to evaluating LAA function in order to determine the risk of cardioembolic complications. In AF, LAA contractility and function are reduced, which often presents with reduced Doppler velocity and LAA dilation (3,4). Therefore, pulse Doppler measurement of the LAA flow velocity is the preferred method for evaluating LAA function. However, research has shown that evaluation of LAA function using the pulse Doppler method alone is not always satisfactory, due to the great variation of blood flow patterns and amplitudes during AF, as well as the shape and velocity of current curve being significantly affected by the location of the sampling volume (5). Recently, Doppler tissue imaging (DTI) has also been used to assess LAA function, and it has good prospects (6-10). However, DTI also has the limitations of being greatly affected by tethering and the translation effects of the heart as well as the angle of acoustic beam, which prevents it from providing a true measurement of myocardial contractility (5). Strain imaging has been found to overcome these limitations, not only because it is superior to DTI in myocardial contractility, but also because myocardial strain analysis can show the level of myocardial deformation (11).
Understanding the changes in the mechanical movement of the LAA in patients with AF can shed light on the formation mechanism of LAA thrombosis. Mechanical damage of the LAA is commonly observed in patients with AF. However, few studies have reported on the characteristics of mechanical damage of the LAA in patients with paroxysmal AF (par-AF) and persistent AF (per-AF), or on the local function of each LAA segment. Therefore, the main purpose of our study was to observe the movement and function characteristics of the LAA in patients with different types of AF using two-dimensional (2D) speckle-tracking transesophageal echocardiography (TEE).
We present the following article in accordance with the STROBE reporting checklist (available at https://qims.amegroups.com/article/view/10.21037/qims-21-942/rc).
Methods
Study population
A cross-sectional, retrospective study was conducted to assess 216 consecutive patients who were diagnosed with AF in the First Affiliated Hospital of Soochow University between January 2019 and January 2020. Par-AF means that AF can be recovered by itself or under intervention within 7 days but will relapse with different frequencies. Per-AF refers to the onset of continuous AF for more than 7 days (12). The inclusion criteria were as follows: patients with non-valvular atrial fibrillation (NVAF) with adequate image quality and complete clinical data. Informed consent was obtained from each patient. Basic information collected about the patients included age, height, weight, and the type of AF (paroxysmal or persistent). Past history of hypertension, diabetes, coronary heart disease, prior stroke, or congenital heart disease was recorded. The exclusion criteria were as follows: patients with congenital heart disease, valvular heart disease, thrombocytopenic purpura, severe mitral regurgitation, or tumor, and patients whose data included low-quality images (Figure 1). This study was conducted in accordance with the Declaration of Helsinki (as revised in 2013) and approved by the Ethics Committee of The Affiliated Hospital of Soochow University (No. 112/2021).
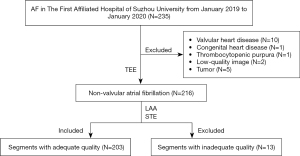
Transthoracic echocardiography
The left atrial diameter (LAD) was measured by a GE VividE95 echocardiograph (GE Vingmed Ultrasound AS, Horten Norway) equipped with an M5S probe on the long-axis section of the left ventricle. The early diastolic flow-velocity peak of the mitral valve (peak E) was measured by pulse Doppler in the apical four-chamber view. DTI was used to measure the early diastolic tissue Doppler velocity of the mitral annulus from septal and lateral (E’sep and E’la). The ratio of E to E’ (E/e’) was then calculated. Simpson’s rule was used to measure the left ventricular ejection fraction (LVEF) in the apical four-chamber view and the apical two-chamber view.
TEE
All patients routinely underwent TEE using a Vivid E95 echocardiography scanner equipped with an M5Sc (1.4–4.6 MHz) probe and a multiplane 6VT (3.0–8.0 MHz) transducer. Lidocaine hydrochloride spray was used for local anesthesia before TEE examinations, and a comprehensive visual assessment of the LAA was performed at the mid-esophageal position by sweeping from 0° to 180°. The left atrial appendage ejection fraction (LAAEF) was measured and calculated using the 2D Simpson’s method. LAAEF = [maximum volume of left atrial appendage (LAA V max) − minimal volume of left atrial appendage (LAA V min)]/(LAA V max) ×100%. The peak empty velocity (PEV) and the peak filling velocity (PFV) were measured by pulse Doppler. The dispersion of the LAA normal strain peak was the standard deviation (SD) of the peak of the positive strain at each segment of the R-R interval. The speckle-tracking echocardiography (STE) system stored 3 to 5 consecutive cardiac cycles in cine loop format, which were used for strain analysis. The analysis relied on an Echo PAC ultrasound workstation (Echo PAC PC Version 203). As the LAA was anterior to anterolateral in position, it was best viewed between 50° and 90° (13). To resolve the problem of beat-to-beat variation in STE measurements, we used the index-beat method (14).
LAA 2D-STE analysis
The edges of the endocardium and epicardium were delineated by offline analysis at the Echo PAC ultrasound workstation in a 2D grayscale dynamic graph between 50° and 90° in the LAA. The starting point of the QRS wave in the electrocardiogram was used as the zero baseline, and the positive strain value of each segment of the LAA was recorded in the strain curve corrected by the R-R interval of the electrocardiogram (Figure 2). The left atrial appendage mechanical dispersion (LAAMD) was defined as the standard deviation (SD) of the peak positive strain at each segment of the R-R interval. Segments with an inadequate quality were excluded. The final analysis involved 203 (94%) LAA segments.
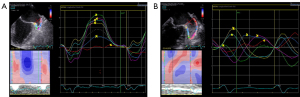
Statistical analysis
SPSS 23.0 (IBM Corp., Armonk, NY, USA) was used for statistical analyses. Continuous variables were described by mean ± standard deviation, and categorical variables were described as percentages. Variables with non-normal distribution were expressed as the median and quartile, i.e., median (P25, P75). The Mann-Whitney U test was used for nonparametric tests. Comparisons between groups were performed using the independent-samples t-test, Mann-Whitney U test, chi-square test, or Fisher’s exact test. Differences were of statistical significance at P<0.05.
Results
Clinical and echocardiographic characteristics of the study population
Compared to the per-AF group, the par-AF group included more women and had a higher use rate of the anticoagulant dabigatran, and these differences were statistically significant (Table 1). There was a higher incidence of heart failure, a higher ratio of previous stroke history, more obvious LAA dilatation, lower LVEF, an increased early diastolic flow-velocity peak (E), a shortened mitral E-wave deceleration time (EDT), increased early diastolic velocity of the mitral annulus, and increased pulmonary artery systolic pressure in the per-AF group than in the par-AF group. All of these parameters were statistically significantly different between the two groups (P<0.05).
Table 1
Variables | Par-AF (n=114) | Per-AF (n=102) | P value |
---|---|---|---|
Sex, female, % | 62.4 | 37.6 | 0.016 |
Age, years | 62.32±9.721 | 63.44±10.427 | 0.413 |
Hypertension, % | 51.6 | 48.4 | 0.391 |
Diabetes, % | 54.5 | 45.5 | 0.521 |
Stroke, % | 31.8 | 68.2 | 0.032 |
Heart failure, % | 10.0 | 90.0 | 0.000 |
Amiodarone, % | 52.7 | 47.3 | 0.511 |
Dabigatran, % | 59.0 | 41.0 | 0.032 |
Rivaroxaban, % | 47.5 | 52.5 | 0.147 |
Propafenone, % | 57.1 | 42.9 | 0.353 |
Warfarin, % | 41.2 | 58.8 | 0.228 |
left atrium, mm | 41.73±4.572 | 47.02±5.091 | 0.000 |
LVEF, % | 62.97±5.716 | 57.11±10.48 | 0.000 |
Cha2ds2vasc | 1.87±1.39 | 2.17±1.59 | 0.144 |
E (cm/s) | 79.02±23.957 | 93.57±22.889 | 0.000 |
EDT (s) | 204.36±52.277 | 172.14±50.76 | 0.000 |
E’(sep) (cm/s) | 7.09±1.94 | 8.43±2.49 | 0.000 |
E’(la) (cm/s) | 9.60±2.98 | 11.86±3.27 | 0.000 |
E/e’ | 9.63±3.309 | 9.88±3.757 | 0.623 |
PASP (mmHg) | 26.72±5.770 | 29.61±8.923 | 0.005 |
Data are expressed as mean ± SD or as %. E’(sep) and E’(lat): early diastolic tissue Doppler velocity of mitral annulus from septal and lateral. E/e’ = ratio of peak early mitral inflow velocity and peak early diastolic mitral annular velocity. LVEF, left ventricular ejection fraction; E, the early diastolic flow velocity peak of mitral valve; EDT, mitral E-wave deceleration time; PASP, pulmonary artery systolic pressure.
TEE characteristics of the study population
Regarding the comparisons of TEE parameters (Table 2), the peak empty velocity (PEV) and peak filling velocity (PFV) of the LAA were lower in patients with per-AF than in patients with par-AF. Furthermore, the ratio of the peak filling velocity of the left atrial appendage (LAAFV) to the peak empty velocity of the left atrial appendage (LAAEV) in the per-AF group was higher, the diameter and depth of the LAA ostium were higher, and the LAAEF was lower than those in the par-AF group (P<0.05). There were also statistically significant differences in partial LAA speckle-tracking parameters between the two groups. In particular, the peak strain on the top segment of the LAA was lower in the per-AF group than in the par-AF group (P<0.01).
Table 2
Variables | Par-AF (n=114) | Per-AF (n=102) | P value |
---|---|---|---|
LAAEV, cm/s | 57.07±25.092 | 31.5±12.430 | 0.000 |
LAAFV, cm/s | 53.0±22.357 | 38.6±16.64 | 0.000 |
LAAFV/LAAEV | 0.97±0.293 | 1.20±0.339 | 0.000 |
LAA ostia, mm | 18.32±3.181 | 20.21±3.494 | 0.000 |
LAA depth, mm | 28.50±5.567 | 31.10±6.856 | 0.003 |
LAAEF, % | 77.4±19.922 | 46.59±19.587 | 0.000 |
LAA PPS (B inside), % | 3.87 (1.99–9.68) | 3.00 (1.00–6.88) | 0.281 |
LAA PPS (M inside), % | 5.70 (2.81–12.66) | 3.38 (1.84–7.97) | 0.098 |
LAA PPS (A inside), % | 16.02 (9.76–24.50) | 11.87 (6.47–20.12) | 0.003 |
LAA PPS (A outside), % | 20.16 (14.16–30.56) | 12.66 (6.66–21.22) | <0.001 |
LAA PPS (M outside), % | 8.36 (3.50–13.56) | 4.97 (2.94–8.53) | 0.000 |
LAA PPS (B outside), % | 6.09 (2.24–10.93) | 4.22 (1.87–7.97) | 0.174 |
Data are expressed as mean ± standard deviation (SD) or as median (IQR). LAAEV, the peak empty velocity of the left atrial appendage; LAAFV, the peak filling velocity of the left atrial appendage; LAA, left atrial appendage; LAAEF, left atrial appendage ejection fraction; LAA PPS, peak positive strain of left atrial appendage; B, basal; M, middle, A, top; Inside, medial wall of the left atrial appendage; Outside, lateral wall of the left atrial appendage.
Clinical and echocardiographic characteristics of the study population about LAAMD
Table 3 details the comparisons of dispersion of the positive strain peak in the LAA. The median LAAMD was 6.28%; patients with LAAMD >6.28% comprised the higher LAAMD group, and those with LAAMD ≤6.28% made up the lower LAAMD group. The proportion of per-AF was higher, the left atrial dilatation was more evident, the filling velocity and emptying velocity of the LAA were lower, the LAAEF was decreased significantly, and the positive peak strain of the middle and top segments of the LAA were decreased visibly in the lower LAAMD group compared with the higher LAAMD group. The difference between the two groups showed statistical significance (P<0.05).
Table 3
Variables | LAAMD (low, n=98) | LAAMD (higher, n=105) | P value |
---|---|---|---|
Sex, female, % | 52.6 | 47.4 | 0.657 |
AF, per-, % | 66.3 | 33.7 | <0.001 |
Hypertension, % | 52.7 | 47.3 | 0.463 |
Diabetes, % | 63.2 | 36.8 | 0.334 |
Stroke, % | 45.0 | 55.0 | 0.814 |
Heart failure, % | 61.1 | 38.9 | 0.458 |
Age, years | 62.91±10.67 | 62.46±9.201 | 0.760 |
Cha2ds2vasc | 2.05±1.454 | 2.00±1.605 | 0.813 |
Left atrium, mm | 45.80±5.656 | 42.85±4.867 | <0.001 |
LVEF, % | 58.58±9.412 | 61.35±7.299 | 0.025 |
LAAEV, cm/s | 38.71±24.39 | 51.62±21.282 | <0.001 |
LAAFV, cm/s | 42.35±20.354 | 51.0±20.599 | 0.004 |
LAA diameter, mm | 19.48±3.19 | 19.14±3.472 | 0.482 |
LAA depth, mm | 30.03±6.946 | 29.41±5.931 | 0.508 |
LAAEF, % | 52.16±25.538 | 70.85±20.741 | 0.000 |
LAA PPS (B inside), % | 3.13 (1.00–6.88) | 4.00 (1.87-9.19) | 0.562 |
LAA PPS (M inside), % | 3.75 (1.56–7.50) | 5.94 (2.97–12.66) | 0.042 |
LAA PPS (A inside), % | 8.91 (5.16–12.38) | 22.31 (15.78–27.75) | 0.000 |
LAA PPS (A outside), % | 10.31 (5.50–14.38) | 25.03 (19.69–31.88) | 0.000 |
LAA PPS (M outside), % | 4.53 (2.62–8.13) | 9.00 (4.22–14.06) | 0.000 |
LAA PPS (B outside), % | 4.50 (2.19–8.63) | 5.81 (2.25–11.09) | 0.562 |
LAAMD (lower): <6.88%. LAAMD (higher): ≥6.88%. Data are expressed as mean ± standard deviation (SD) and % or as median (interquartile range). LAAMD, left atrial appendage mechanical dispersion; LAAEV, the peak empty velocity of the left atrial appendage; LAAFV, the peak filling velocity of the left atrial appendage; LAA, left atrial appendage; LAAEF, left atrial appendage ejection fraction; LAA PPS, peak positive strain of the left atrial appendage; B, basal; M, middle; A, top; Inside, medial wall of the left atrial appendage; Outside, lateral wall of the left atrial appendage.
The peak positive strain of each segment of the LAA in different AF subtypes
Figure 3 shows that the peak positive strain of each LAA segment differed between the par-AF group and the per-AF group. In the basal and the top segments of the LAA, both medial and lateral, the per-AF group had a lower strain value than the par-AF group, and the differences were statistically significant (P=0.018, P=0.044, P=0.044, P<0.001, respectively). The middle segment showed that strain value, in the inside group, the per-AF was lower than the par-AF, and the difference was statistically significant (P=0.014). In the outside group, per-AF was lower than par-AF, the difference was not statistically significant (P>0.05). The peak positive strain of each segment of the LAA differed between the low LAAMD group and the high LAAMD group; in the middle and the top of segments of the LAA, both inside and outside, the differences were statistically significant (P=0.001 and P<0.001, respectively). The dispersion of the peak systolic strain of the LAA differed between the par-AF group and the per-AF group (P=0.000), with the peak positive strain of the per-AF group being more concentrated.
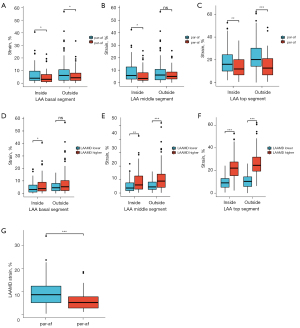
Discussion
The development of ultrasonic technology has enabled researchers to better understand the structure and function of the LAA. The LAA is a finger-like extension originating from the main body of the left atrium, which can actively relax and contract (15). The compliance and blood-storing ability of LAA are much greater in the LAA than in other areas of the left atrium (16). The LAA is the most common site of thrombosis in patients with AF due to its complex structure and trabecular muscles. The contractility of the LAA decreases in the event of AF, which prevents blood from being effectively eliminated, causing blood retention (16). Results shown in an ultrasound are characterized by decreases in LAAEV and LAAEF. Reduced LAAFV was significantly associated with a high prevalence of non-paroxysmal AF and reduced LVEF. Fukuhara et al. (17) showed that reduced LAAFV was not correlated with significant differences in heart rate. In our study, patients with per-AF had lower LAAEF than the par-AF group. The LAA has a distinct systolic pattern; in the first passive phase in early diastole, there is a biphasic empty pattern, and in the second active phase in left atrium contraction, there is a prominent monophasic filling pattern. This pattern in AF is characterized by rapid alternation of emptying and filling at a low rate (18).
The left atrium is the cardiac structure most closely related to AF. There is causality between AF and left atrial structural remodeling, which mainly manifests as an increase in the left atrial inner diameter. AF can lead to atrial structural remodeling and myocardial fibrosis (19). Long-term AF further results in increased stiffness, an enlarged inner diameter, and histological changes in the thin-walled left atrium. In our study, the left atrial inner diameter was significantly increased in patients with per-AF. Additionally, we observed that the early diastolic flow-velocity peak (E) was increased, the EDT was shortened, and the E/e’ was increased in the per-AF group, which may indicate elevated left atrial pressure. This observation suggests that AF may have enhanced left atrial remodeling by expanding the left atrium, leading to the increase in left atrial pressure and ultimately affecting the flow velocity of the LAA.
With the increase in left atrial pressure, the LAA gradually enlarges, which is a compensatory adjustment to relieve increased left atrial pressure. This enlargement of the LAA also constitutes an important anatomical structural basis for the LAA having reduced mechanical function and blood flow stasis, which lead to thrombosis. It is common to see LAA remodeling in the pathophysiology of AF, and the orifice and depth of the LAA can also serve as indicators of LAA remodeling. Patti et al. (20) found that the structure of the LAA was found to be negatively correlated with its function. Currently, the PEV and LAAEF of the LAA are frequently used as objective and preferred indicators for evaluating LAA function, because LAA function is mainly reflected in the systolic and diastolic capacity of the LAA. In patients with AF, LAA function ranges from normal to low, with varying degrees of loss. In general, severe LAA remodeling leads to the deterioration of LAA mechanical function (loss of contractility) and results in a static pouch-like change presented in the LAA, which causes blood flow stasis and thrombosis. In our study, patients with per-AF had significant LAA dilation, a decreased overall ejection fraction, decreased LAAEV, and a diffusely decreased positive strain peak compared with the par-AF group. These results also indicated a relationship between AF duration and LAAMD: the longer the duration of AF, the more obvious the reduction in LAA deformability, and contractility resented a diffuse decline.
The normal flow cycle begins just after the mitral valve opens, with an early diastolic forward flow (LAA emptying), which is determined by the intracavitary suction and ventricular filling (5). Left ventricular dilation which occurs during the diastolic filling period may further facilitate emptying of the LAA by compressing the free ventricular wall and immobilizing the inferior medial wall of the LAA between the pericardium (21). The decreased ability of the LAA to empty can be attributed to the reduced diastolic function of the left ventricle, and patients with AF have limited filling. If the left ventricular filling pressure or left atrial pressure increases, it will affect the blood flow velocity of the LAA. The top segment of the LAA has a longer extension and it appears to be less compressed by the free ventricular wall. Therefore, it is possible that when the disease progresses for a certain period of time, the top segment of the LAA sustains mechanical damage, and the contractility significantly and actively decreases. In our study, the peak positive strain of the top segment of the LAA in patients with per-AF decreased more significantly than it did in patients with par-AF, as did the LAAEF. In addition, the contractility of the whole ventricular wall of the LAA generally decreased, and the peak positive strain decreased centrally in patients with per-AF. Previous research by Shirani et al. (22) on pathological tissue from the LAA of patients with AF found that the most fibrotic LAA was at the base of the LAA, whereas the middle and top of the LAA sequentially decreased. In our study, the deformability of the basal segment of the LAA was lower than that of the remaining myocardium wall in both groups, which may be related to the degree of LAA fibrosis. However, the study found that patients with par-AF had a relatively high strain peak and great dispersion, which may be caused by retention of the deformability of the local ventricular wall of the LAA in such patients.
Ma et al. (23) found that the expression of the M2 receptor was higher in patients with AF than in the non-AF group. TGF-β1 is a key regulator of fibrosis that enhances collagen synthesis (24). The expression of TGF-β1 and CTGF in LAA tissues is correlated with serum anti-M2-R levels and the upregulation of the M2 receptor and TGF-β1 expression in the LAA of patients with AF (23). Persistent AF has been reported to result in fibroblast accumulation in several regions, particularly in the LAA (25). The LAA acts as a buffer by increasing compliance to reduce the increased pressure in the left atrium (26) and prevent the long-term left atrial remodeling that leads to AF (27). If compliance becomes poor, it may contribute to further remodeling of the left atrium, the persistence of AF and the need for antiarrhythmic drugs, and a poor response to radiofrequency ablation. This may also be the reason why compliance of the LAA in per-AF is worse than that of par-AF. There may be fibrotic changes in the LAA, and the degree of fibrosis is related to the duration of AF.
TEE is an invasive examination method. Therefore, it is difficult to invite healthy participants with no cardiomyopathy to take part in an LAA function examination. Previous research has shown that patients with per-AF and embolic events have significantly worse LAA strain and a worse strain rate than patients without embolization (28). Although we observed no difference in the frequency of stroke between the high and low LAAMD groups, but this requires a larger sample size with a longer follow-up time.
Limitations
Our study had several important limitations. First, it was a single-center study with a small sample size. Additionally, the study was a retrospective analysis. Also, although we used 2D echocardiography to measure the LAAEF, three-dimensional echocardiography can provide a more accurate measurement. In view of the factors mentioned above, it is necessary to conduct further studies with larger participant cohorts. In future, baseline strain measurements should be assessed before stroke onset, but this will require a larger sample size with a longer follow-up time.
Conclusions
The mechanical function of the LAA can be quantified by 2D speckle-tracking TEE in patients with non-valvular AF. The deformability of the LAA is decreased diffusely in patients with per-AF, especially in the top segment of the LAA. In these patients, the LAA is also significantly enlarged, and its filling velocity and emptying velocity are decreased. Compliance with LAA is worse in patients with per-AF than in those with par-AF.
Acknowledgments
The authors would like to thank all the patients who participated in this study.
Funding: None.
Footnote
Reporting Checklist: The authors have completed the STROBE reporting checklist (available at https://qims.amegroups.com/article/view/10.21037/qims-21-942/rc).
Conflicts of Interest: All authors have completed the ICMJE uniform disclosure form (available at https://qims.amegroups.com/article/view/10.21037/qims-21-942/coif). The authors have no conflicts of interest to declare.
Ethical Statement: The authors are accountable for all aspects of the work in ensuring that questions related to the accuracy or integrity of any part of the work are appropriately investigated and resolved. The study was conducted in accordance with the Declaration of Helsinki (as revised in 2013) and approved by the Ethics Committee of The Affiliated Hospital of Soochow University (No. 112/2021) and informed consent was taken from all the patients.
Open Access Statement: This is an Open Access article distributed in accordance with the Creative Commons Attribution-NonCommercial-NoDerivs 4.0 International License (CC BY-NC-ND 4.0), which permits the non-commercial replication and distribution of the article with the strict proviso that no changes or edits are made and the original work is properly cited (including links to both the formal publication through the relevant DOI and the license). See: https://creativecommons.org/licenses/by-nc-nd/4.0/.
References
- Seet RC, Friedman PA, Rabinstein AA. Prolonged rhythm monitoring for the detection of occult paroxysmal atrial fibrillation in ischemic stroke of unknown cause. Circulation 2011;124:477-86. [Crossref] [PubMed]
- Beigel R, Wunderlich NC, Ho SY, Arsanjani R, Siegel RJ. The left atrial appendage: anatomy, function, and noninvasive evaluation. JACC Cardiovasc Imaging 2014;7:1251-65. [Crossref] [PubMed]
- Pollick C, Taylor D. Assessment of left atrial appendage function by transesophageal echocardiography. Implications for the development of thrombus. Circulation 1991;84:223-31. [Crossref] [PubMed]
- Nucifora G, Faletra FF, Regoli F, Pasotti E, Pedrazzini G, Moccetti T, Auricchio A. Evaluation of the left atrial appendage with real-time 3-dimensional transesophageal echocardiography: implications for catheter-based left atrial appendage closure. Circ Cardiovasc Imaging 2011;4:514-23. [Crossref] [PubMed]
- Bansal M, Kasliwal RR. Echocardiography for left atrial appendage structure and function. Indian Heart J 2012;64:469-75. [Crossref] [PubMed]
- Tenekecioğlu E, Karabulut A, Yilmaz M. Comparison of tissue Doppler dynamics with Doppler flow in evaluating left atrial appendage function by transesophageal echocardiography in prehypertensive and hypertensive patients. Echocardiography 2010;27:677-86. [Crossref] [PubMed]
- Bauer F, Verdonck A, Schuster I, Tron C, Eltchaninoff H, Cribier A, Derumeaux G. Left atrial appendage function analyzed by tissue Doppler imaging in mitral stenosis: effect of afterload reduction after mitral valve commissurotomy. J Am Soc Echocardiogr 2005;18:934-9. [Crossref] [PubMed]
- Cayli M, Acartürk E, Demir M, Kanadaşi M. Systolic tissue velocity is a useful echocardiographic parameter in assessment of left atrial appendage function in patients with mitral stenosis. Echocardiography 2007;24:816-22. [Crossref] [PubMed]
- Vijayvergiya R, Sharma R, Shetty R, Subramaniyan A, Karna S, Chongtham D. Effect of percutaneous transvenous mitral commissurotomy on left atrial appendage function: an immediate and 6-month follow-up transesophageal Doppler study. J Am Soc Echocardiogr 2011;24:1260-7. [Crossref] [PubMed]
- Yakar Tuluce S, Kayikcioglu M, Tuluce K, Yilmaz MG, Ozdogan O, Aydın M, Hasdemir C. Assessment of left atrial appendage function during sinus rhythm in patients with hypertrophic cardiomyopathy: transesophageal echocardiography and tissue doppler study. J Am Soc Echocardiogr 2010;23:1207-16. [Crossref] [PubMed]
- Kaya EB, Tokgözoglu L, Aytemir K, Kocabas U, Tülümen E, Deveci OS, Köse S, Kabakçi G, Nazli N, Ozkutlu H, Oto A. Atrial myocardial deformation properties are temporarily reduced after cardioversion for atrial fibrillation and correlate well with left atrial appendage function. Eur J Echocardiogr 2008;9:472-7. [PubMed]
- January CT, Wann LS, Alpert JS, Calkins H, Cigarroa JE, Cleveland JC Jr, Conti JB, Ellinor PT, Ezekowitz MD, Field ME, Murray KT, Sacco RL, Stevenson WG, Tchou PJ, Tracy CM, Yancy CWACC/AHA Task Force Members. 2014 AHA/ACC/HRS guideline for the management of patients with atrial fibrillation: executive summary: a report of the American College of Cardiology/American Heart Association Task Force on practice guidelines and the Heart Rhythm Society. Circulation 2014;130:2071-104. [Crossref] [PubMed]
- Agmon Y, Khandheria BK, Gentile F, Seward JB. Echocardiographic assessment of the left atrial appendage. J Am Coll Cardiol 1999;34:1867-77. [Crossref] [PubMed]
- Kusunose K, Yamada H, Nishio S, Tomita N, Hotchi J, Bando M, Niki T, Yamaguchi K, Taketani Y, Iwase T, Soeki T, Wakatsuki T, Sata M. Index-beat assessment of left ventricular systolic and diastolic function during atrial fibrillation using myocardial strain and strain rate. J Am Soc Echocardiogr 2012;25:953-9. [Crossref] [PubMed]
- Delgado V, Di Biase L, Leung M, Romero J, Tops LF, Casadei B, Marrouche N, Bax JJ. Structure and Function of the Left Atrium and Left Atrial Appendage: AF and Stroke Implications. J Am Coll Cardiol 2017;70:3157-72. [Crossref] [PubMed]
- Li J, Li Q, Alqahtany FS, Algahtani FH, Kim HJ, Li Y, Ock Kim Y. Evaluating the novel parameters for assessing the LAA function and thrombus formation with nonvalvular atrial fibrillation. Saudi J Biol Sci 2021;28:560-5. [Crossref] [PubMed]
- Fukuhara E, Mine T, Kishima H, Ishihara M. Predictors for reduced flow velocity in left atrial appendage during sinus rhythm in patients with atrial fibrillation. Heart Vessels 2021;36:393-400. [Crossref] [PubMed]
- Regazzoli D, Ancona F, Trevisi N, Guarracini F, Radinovic A, Oppizzi M, Agricola E, Marzi A, Sora NC, Della Bella P, Mazzone P. Left Atrial Appendage: Physiology, Pathology, and Role as a Therapeutic Target. Biomed Res Int 2015;2015:205013. [Crossref] [PubMed]
- Chen L, Zhang C, Wang J, Guo L, Wang X, Liu F, Li X, Zhao Y. Left atrial strain measured by 4D Auto LAQ echocardiography is significantly correlated with high risk of thromboembolism in patients with non-valvular atrial fibrillation. Quant Imaging Med Surg 2021;11:3920-31. [Crossref] [PubMed]
- Patti G, Pengo V, Marcucci R, Cirillo P, Renda G, Santilli F, et al. The left atrial appendage: from embryology to prevention of thromboembolism. Eur Heart J 2017;38:877-87. [PubMed]
- Akosah KO, Funai JT, Porter TR, Jesse RL, Mohanty PK. Left atrial appendage contractile function in atrial fibrillation. Influence of heart rate and cardioversion to sinus rhythm. Chest 1995;107:690-6. [Crossref] [PubMed]
- Shirani J, Alaeddini J. Structural remodeling of the left atrial appendage in patients with chronic non-valvular atrial fibrillation: Implications for thrombus formation, systemic embolism, and assessment by transesophageal echocardiography. Cardiovasc Pathol 2000;9:95-101. [Crossref] [PubMed]
- Ma G, Wu X, Zeng L, Jin J, Liu X, Zhang J, Zhang L. Association of Autoantibodies against M2-Muscarinic Acetylcholine Receptor with Atrial Fibrosis in Atrial Fibrillation Patients. Cardiol Res Pract 2019;2019:8271871. [Crossref] [PubMed]
- Thanigaimani S, Lau DH, Agbaedeng T, Elliott AD, Mahajan R, Sanders P. Molecular mechanisms of atrial fibrosis: implications for the clinic. Expert Rev Cardiovasc Ther 2017;15:247-56. [Crossref] [PubMed]
- Saljic A, Friederike Fenner M, Winters J, Flethøj M, Eggert Eggertsen C, Carstensen H, Dalgas Nissen S, Melis Hesselkilde E, van Hunnik A, Schotten U, Sørensen U, Jespersen T, Verheule S, Buhl R. Increased fibroblast accumulation in the equine heart following persistent atrial fibrillation. Int J Cardiol Heart Vasc 2021;35:100842. [Crossref] [PubMed]
- Davis CA 3rd, Rembert JC, Greenfield JC Jr. Compliance of left atrium with and without left atrium appendage. Am J Physiol 1990;259:H1006-8. [PubMed]
- Hoit BD, Walsh RA. Regional atrial distensibility. Am J Physiol 1992;262:H1356-60. [PubMed]
- Jankajova M, Kubikova L, Valocik G, Candik P, Mitro P, Kurecko M, Sabol F, Kolesar A, Kubikova M, Vachalcova M, Dvoroznakova M. Left atrial appendage strain rate is associated with documented thromboembolism in nonvalvular atrial fibrillation. Wien Klin Wochenschr 2019;131:156-64. [Crossref] [PubMed]