Magnetic resonance imaging using heparin-coated superparamagnetic iron oxide nanoparticles for cell tracking in vivo
Abstract
Magnetic resonance imaging (MRI) is a tremendous modality for noninvasive cell tracking. To this end, superparamagnetic iron oxide (SPIO), one of the MRI contrast agents, should be labeled to the cells before transplantation. Currently, cellular labelling with SPIOs such as Feridex and Resovist is generally carried out through their engulfment into cytosol via endocytosis. However, the labelling efficacy via endocytosis is relatively low due to their non-specific random engulfment and degradation in the cytosol. To overcome these limitations, transfection agents such as poly-L-lysine and lipofectamine are complexed with SPIOs and treated to the cells. However, these strategies should be optimized due to the cytotoxicity of transfection agents themselves. Recently, there were developments of chemical conjugation of SPIOs onto cellular membrane. To this end, the surface of SPIOs was coated with heparin polysaccharide and chemically conjugated with collagen matrix layer of cell surface by using linker polymer, which was stably maintained in vivo. This new remedy can overcome the limitations of cell labelling via endocytosis. Collectively, these strategies could be applied for noninvasive imaging of MRI after cell transplantation in vivo.
Key words
Superparamagnetic iron oxide; MRI; cellular endocytosis; surface modification; transplantation
Introduction
Visualization of transplanted cells in the body is very important for cell-based therapy. Currently, there are many modalities such as positron-emission tomography (PET), ultrasonography, computerized tomography (CT), and magnetic resonance imaging (MRI). Particularly, MRI is a good modality for cell tracking because it has the high-resolution power to track the tiny cells in the body and is safe to patients (1). For operation with MRI, MR contrast agents are generally used to provide the regional contrast of transplanted cells. To this end, SPIO (superparamagnetic iron oxide) nanoparticles are typically utilized, which has various applications such as targeted drug or gene delivery, biosensor, tissue engineering and magnetic transfection (2-4).
For in vivo cell tracking, the typical method is that SPIO nanoparticles such as Feridex and Resovist are delivered into cytosol of cells. However, the cellular engulfment of Feridex and Resovist are randomly occurred because there is no driving force, thereby showing irregular engulfment efficacy. Therefore, recently many types of SPIO nanoparticles have been developed in experimental study stages (2,5). Here we highlight a new SPIO nanoparticles for cell trafficking.
Heparin-coated SPIO (HSPIO) as a new MRI contrast agent
As a T2 contrast agent, SPIOs can be divided into three categories according to their hydrodynamic diameter: Oral SPIO, 300 nm -3.5 μm; Standard SPIO, 50-150 nm; and Ultrasmall SPIO (USPIO), <50 nm (6). These SPIOs tend to be aggregated because of their surface-to-volume ratio and dipole-dipole interaction, thereby reducing intrinsic superparamagnetic property that relatively low r2 relaxivity. To inhibit self-aggregation of SPIOs, the surface of SPIO nanoparticles is coated with polysaccharide or synthetic polymers. Surface modification of SPIOs can privilege several advantages such as prevention of agglomeration of the iron oxide core, colloidal stability, water solubility, biocompatibility and nonspecific adsorption to cells. Additionally, the coated materials can provide chemical handles for the conjugation of drug molecules, targeting ligands, and reporter moieties. Also, there are efforts to improve magnetic property and increase relaxivity by controlling the composition (7,8), aggregation (9), and oxidation state (10) of SPIO nanoparticles since r2 relaxivity is directly related to the magnetic properties of the nanoparticles.
Heparin, molecular weight between 3 and 30 kDa, is composed of sulfated glycosaminoglycan and widely used as an anticoagulant medication. Recently, heparin-coated SPIO (HSPIO) has been developed due to specific function of heparin molecule (11-14). The HSPIOs are synthesized in alkaline co-precipitation process Fe(II) and Fe(III) precursors which is similar to dextran-coated SPIO, i.e., Feridex and Resovist (Figure 1). Diameter of the crystal core is ~10 nm and hydrodynamic nanoparticle diameter is 60-80 nm. Compared to Feridex and Resovist, HSPIOs have a narrow size distribution with their branch-like structure, meaning that themselves are not aggregated in the solution (15). Its r1 and r2 relaxivities are 9.4 and 170.7 mM-1·sec-1, respectively, and the r2/r1 ratio is 18.2, indicating that HSPIO can be strongly used as a T2 MRI contrast agent, compared to Feridex and Resovist. The reasons are that higher r2/r1 ratio of HSPIO may be attributed to higher hydrodynamic size of heparin itself and more effective magnetic relaxation from the proton spins around HSPIO due to the interactions between water molecules and multiple sulfate/carboxylic groups of heparin polysaccharide. Table 1 summarize the properties of Feridex, Resovist and HSPIO.
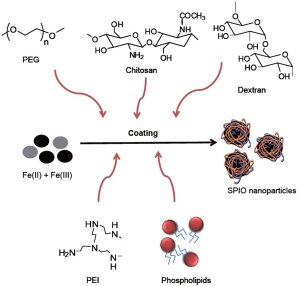
Table 1 Comparison of MR contrast agents for cellular labeling | |||
Feridex® | Resovist® | Heparin-SPIO | |
Coating polymer | Dextran T-10 | Carboxydextran | Heparin |
Core diameter (nm) | 4.8-5.6 | 4-13 | 10 |
Hydrodynamic diameter (nm) | 120-180 | 60 | 60-80 |
Central moiety | Fe2+, Fe3+ | Fe2+ | Fe2+, Fe3+ |
MR relaxivity | r1 =40.0, r2 =160 | r1 =25.4, r2 =151 | r1 =9.4, r2 =170.7 |
Surface charge | Negative | Negative | Negative |
In vivo MR imaging of human mesenchymal stem cells using HSPIO
Mesenchymal stem cells (MSCs) are multipotent stromal cells that can differentiate into a variety of cell types and have emerged as a promising cell source in tissue engineering due to their large capacity for self-renewal while maintain their multipotency (16-18). It is very important to evaluate their therapeutic roles in regeneration of damaged target tissue by in vivo MRI monitoring their survival, growth, and migration of transplanted stem cells. However, it is reported that stem cells rarely engulf SPIO nanoparticles due to their limited endocytosis and phagocytosis activities (19). When the HSPIOs were treated, MSCs could engulf ~4-fold larger amount of HSPIOs into cytosol, when compared to Feridex treatment (15). For the labelling of stem cells, Feridex was treated to stem cells with long treatment time (>24 h) and high concentration (>50 μg/mL). Although these conditions were applied to stem cells, the amount of the engulfed iron had wide range (1.47-17.9 pg Fe/cell) (20). On the other hand, HSPIOs can easily entered stem cells, although they were exposed to stem cells for 1 h incubation. Other previous studies demonstrated that hydrophilic heparin coated onto the surface of biomaterials could facilitate cell adhesion to the surface due to the enhanced hydrophilicity (21,22). HSPIOs consistently show the increased hydrophilicity and enhanced cellular uptake. Moreover, HSPIOs labeled into MSCs did not affect the viability and proliferation activity of MSCs. In addition, intracellular HSPIOs did not effect the osteogenic and adipogenic differentiation of MSCs when their differentiation was induced by the differentiation medium (15). These results demonstrated that HSPIOs did not adversely influence the native metabolic activities or phenotypes including their proliferation and differentiation capacities of stem cells. In addition, to confirm in vivo cellular tracking using HSPIOs, HSPIO-labeled MSCs were transplanted into the renal subcapsular membrane of mice and visualized with T2-weighted MRI. They could be visualized with strong negative intensity during more than one month. Interestingly, the MRI intensity was very sensitive although marginal cell mass of MSCs (2×105 cells/ mouse) was transplanted when compared with general essential stem cells (~100-fold difference). Therefore, these results indicated that HSPIOs could be used with high sensitivity to monitor a marginal cell mass of MSCs.
In vivo imaging of surface-labeled pancreatic islets with HSPIOs
Type 1 diabetes mellitus is occurred by autoreactive T cells, which induce autoimmune destruction of insulin secreting pancreatic islets in the pancreas. Transplantation of pancreatic islets donated from human cadavers is a promising method to cure this disease (23,24). However, most transplanted islets are easily rejected because of graft immune reactions. In this circumstance, physicians use several kinds of immunosuppressive drugs for attenuating immune reactions after islet transplantation. However, the administration regimen of immunosuppressive drugs should be carefully regulated because of severe adverse effects of immunosuppressive drugs. Currently, many hospitals and institutes are following ‘Edmonton Protocol’ for surviving human islet transplants (23). However, this Edmonton protocol does not completely protect transplanted islets 2 years after islet transplantation. Therefore, noninvasive monitoring transplanted islets will help to control graft immune reaction. To this end, pancreatic islets have been labeled with Feridex via endocytosis pathway (1,25-28). For optimal SPIO uptake into islets, there needed several validation strategies: (I) the lack of toxicity toward the islets; (II) the appropriate iron uptake; (III) the rapid clearance of the agent from the environment after cell destruction; (IV) the correlation between the radiologic images and the reality (29).
Feridex uptake into islets has many problems such as random labelling into islets and possibility of cellular toxicity in the cytoplasm when used with transfection agents. To overcome these limitations, the surface modification of islets using HSPIOs, chemically conjugated onto islet surface through polyethylene glycol (PEG) linker, can be one of the solutions (14) (Figure 2). This surface modification reaction is mediated with heterobifunctional PEG polymer, N-hydroxysuccinimide ester (NHS)-PEG-maleimide, biocompatible polymer for anchoring HSPIOs. The active NHS of PEG polymer is able to react with amine group of collagen layer of islet surface, while maleimide of PEG polymer can react with sulfhydryl group of HSPIO. To this end, heparin polysaccharide should be chemically modified to give sulfhydryl group in advance. Compared to Feridex uptake islets, ‘all’ islets are covered with HSPIOs onto islet surface, and with no damage to the viability and functionality of insulin secretion of the islets. When the islets surface-labeled with HSPIOs were transplanted into mouse left renal subcapsular membrane, their hypointensity in MR image was strongly visualized and the MR signal was retained for more than 30 days. Furthermore, the MR signal intensity was proportional to the transplanted number of islets, indicating that surface-labeling strategy to islets can be used for quantitative analysis of transplanted islets without noninvasive methods.
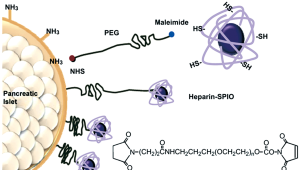
Conclusions
MRI is an useful imaging modality for noninvasive cell tracking because of its high spatial resolution and excellent image quality. Practically, the cell mass to be transplanted is relatively very tiny in the body. Currently, there are developments of several kinds of MR contrast agents, i.e., Feridex, Resovist, and Heparin-coated SPIO, for noninvasive cell tracking in the body. These efforts must overcome the limitations of MRI and contribute to clinical trials of cell-based therapy.
Acknowledgements
This work was supported by the National Research Foundation of Korea (NRF) grant funded by the Ministry of Education, Science and Technology in Republic of Korea (grant no. 2009-0081879, 2010-0002994, 2012-012042).
Disclosure: The authors declare no conflict of interest.
References
- Toso C, Zaidi H, Morel P, et al. Positron-emission tomography imaging of early events after transplantation of islets of Langerhans. Transplantation 2005;79:353-5.
- McCarthy JR, Weissleder R. Multifunctional magnetic nanoparticles for targeted imaging and therapy. Adv Drug Deliv Rev 2008;60:1241-51.
- Salgueirino-Maceira V, Correa-Duarte MA. Increasing the complexity of magnetic core/shell structured nanocomposites for biological applications. Adv Mater 2007;19:4131-44.
- Gupta AK, Naregalkar RR, Vaidya VD, et al. Recent advances on surface engineering of magnetic iron oxide nanoparticles and their biomedical applications. Nanomedicine (Lond) 2007;2:23-39.
- Sun C, Lee JS, Zhang M. Magnetic nanoparticles in MR imaging and drug delivery. Adv Drug Deliv Rev 2008;60:1252-65.
- Elias A, Tsourkas A. Imaging circulating cells and lymphoid tissues with iron oxide nanoparticles. Hematology Am Soc Hematol Educ Program 2009:720-6.
- Seo WS, Lee JH, Sun XM, et al. FeCo/graphitic-shell nanocrystals as advanced magnetic-resonance-imaging and near-infrared agents. Nat Med 2006;5:971-6.
- Lee JH, Huh YM, Jun Y, et al. Artificially engineered magnetic nanoparticles for ultra-sensitive molecular imaging. Nat Med 2007;13:95-9.
- Perez JM, Josephson L, O’Loughlin T, et al. Magnetic relaxation switches capable of sensing molecular interactions. Nat Biotechnol 2002;20:816-20.
- Lee H, Yoon TJ, Weissleder R. Ultrasensitive Detection of Bacteria Using Core-Shell Nanoparticles and an NMR-Filter System. Angew Chem Int Ed Engl 2009;48:5657-60.
- Sjogren CE, Johansson C, Naevestad A, et al. Crystal size and properties of superparamagnetic iron oxide (SPIO) particles. Magn Reson Imaging 1997;15:55-67.
- Lee DY. Highly Effective T2 MR Contrast Agent Based on Heparinized Superparamagnetic Iron Oxide Nanoparticles. Macromolecular Research 2011;19:843-7.
- Jung MJ, Ha YE, Lee DY. Heparin-coated superparamagnetic iron oxide nanoparticles as highly effective MRI contrast agent for cell labeling. J Control Release 2011;152:e214-5.
- Jung MJ, Lee SS, Hwang YH, et al. MRI of transplanted surface-labeled pancreatic islets with heparinized superparamagnetic iron oxide nanoparticles. Biomaterials 2011;32:9391-400.
- Lee JH, Jung MJ, Hwang YH, et al. Heparin-coated superparamagnetic iron oxide for in vivo MR imaging of human MSCs. Biomaterials 2012;33:4861-71.
- Janssens S, Dubois C, Bogaert J, et al. Autologous bone marrow-derived stem-cell transfer in patients with ST-segment elevation myocardial infarction: double-blind, randomised controlled trial. Lancet 2006;367:113-21.
- Lindvall O, Kokaia Z. Stem cells for the treatment of neurological disorders. Nature 2006;441:1094-6.
- Reddi AH, Becerra J, Andrades JA. Nanomaterials and Hydrogel Scaffolds for Articular Cartilage Regeneration. Tissue Eng Part B Rev 2011;17:301-5.
- Reddy AM, Kwak BK, Shim HJ, et al. In vivo Tracking of Mesenchymal Stem Cells Labeled with a Novel Chitosan-coated Superparamagnetic Iron Oxide Nanoparticles using 3.0T MRI. J Korean Med Sci 2010;25:211-9.
- Arbab AS, Yocum GT, Kalish H, et al. Efficient magnetic cell labeling with protamine sulfate complexed to ferumoxides for cellular MRI. Blood 2004;104:1217-23.
- Moon HJ, Yun YP, Han CW, et al. Effect of heparin and alendronate coating on titanium surfaces on inhibition of osteoclast and enhancement of osteoblast function. Biochem Biophys Res Commun 2011;413:194-200.
- Shen H, Hu X, Yang F, et al. Cell affinity for bFGF immobilized heparin-containing poly(lactide-co-glycolide) scaffolds. Biomaterials 2011;32:3404-12.
- Shapiro AM, Lakey JR, Ryan EA, et al. Islet transplantation in seven patients with type 1 diabetes mellitus using a glucocorticoid-free immunosuppressive regimen. N Engl J Med 2000;343:230-8.
- Shapiro AM, Ricordi C, Hering B. Edmonton’s islet success has indeed been replicated elsewhere. Lancet 2003;362:1242.
- Evgenov NV, Medarova Z, Dai GP, et al. In vivo imaging of islet transplantation. Nat Med 2006;12:144-8.
- Evgenov NV, Medarova Z, Pratt J, et al. In vivo imaging of immune rejection in transplanted pancreatic islets. Diabetes 2006;55:2419-28.
- Saudek F, Brogren CH, Manohar S. Imaging the Beta-cell mass: why and how. Rev Diabet Stud 2008;5:6-12.
- Toso C, Vallee JP, Morel P, et al. Clinical magnetic resonance imaging of pancreatic islet grafts after iron nanoparticle labeling. Am J Transplant 2008;8:701-6.
- Ris F, Lepetit-Coiffe M, Meda P, et al. Assessment of human islet labeling with clinical grade iron nanoparticles prior to transplantation for graft monitoring by MRI. Cell Transplant 2010;19:1573-85.