Quantitative analysis of epicardial fat volume: effects of scanning protocol and reproducibility of measurements in non-contrast cardiac CT vs. coronary CT angiography
Introduction
Several studies have focused on the role of the mediastinal (intra- and extra-pericardial) fat in the pathogenesis of cardiovascular disease (CVD), due to the direct contact with the coronary vessels (1,2). Indeed, in case of excess of cardiac fat deposits the delivery of free fatty acids increases expression of the molecules promoting inflammation, which may, in turn, accelerate the atherosclerosis process (3). Although measurements of abdominal visceral fat would be the real effective CVD risk predictor, a standardized quantitative method of measurement is not used in clinical practice. In a recent study it was reported that epicardial fat volume (EFV) was the best predictor for angiographic disease severity compared to waist circumference or visceral abdominal fat volume (4). Furthermore, it has been demonstrated that vascular aging and subclinical atherosclerosis, as revealed by carotid stiffness and intima-media thickness, are related to epicardial fat thickness better than waist circumference in hypertensive patients (5). Even in non-obese patients epicardial fat may be more related to the development of coronary atherosclerosis than the traditional cardiovascular risk factors and abdominal visceral adipose tissue (6). Several imaging techniques such as echocardiography, computed tomography (CT) and magnetic resonance (MR) (7-9) have been used to measure intra- and extra-pericardial fat. The high spatial resolution of the CT may allow an accurate measurement and quantification of EFV, but specific applications have not been developed until now.
The purposes of the study were to evaluate the performance and inter- and intra-observer variability of a computerized method for the quantitative analysis of EFV and epicardial fat density (EFD) from images obtained by coronary calcium scan or non-contrast cardiac CT (NCT) and coronary CT angiography (coronary CTA).
Methods
Patient population
Our study was a retrospective analysis and included a set of 30 patients (mean age =61±12.5 years, 73% male, mean body mass index (BMI) =25.9±6.3 kg/m2) referred to our Institution for suspected CAD between April 2010 and February 2011 (Table 1), who underwent NCT scan for calcium score evaluation followed by coronary CTA for assessment of coronary arteries patency. Clinical and biochemical analysis were collected. All patients gave written informed consent for retrospective use of their data.
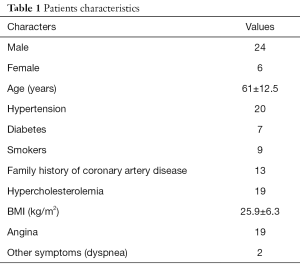
Full table
Cardiac CT
The images were acquired using a multidetector row CT (MDCT, LightSpeed VCT 64, GE Healthcare, Milwaukee, WI, USA). The following parameters were used for coronary calcium scan: detector collimation =2.5 mm, reconstruction interval =2.5 mm, detector coverage =20 mm, (8 detectors × 2.5 mm), gantry rotation time =0.35 ms, tube current =300 mA (automated modulated), and voltage =100 kV, retrospective ECG gating.
For coronary CTA the following parameters and techniques were used: retrospective ECG gating with spiral technique, 64 channel detectors along the z-axis, scan FOV 15–21 cm (depend on the patient size) from the ascending aorta to the level of the diaphragm, matrix 512×512, detector collimation 0.625 mm; reconstruction 0.6×0.6 mm, range of helical pitch 0.18–0.24, gantry rotation time 0.35 ms, tube current range =250–600 mA, depending on patient size and with automated modulation of the kV based on the BMI, 100 or 120 kV. Nonionic iodinated contrast medium (Iomeprol 400, Bracco Imaging Italia s.r.l.) was injected via a peripheral vein with a triphasic protocol using a programmable injector (Nemoto Dual Shot Injector, Nemoto Kyorindo Co. Ltd., Japan) with a two-way syringe system. In the first phase, a rapid injection of contrast medium (8 mL/sec) was performed. In the second phase, 10 mL of contrast at 1 mL/sec were injected simultaneously with 25 mL of saline at 2.5 mL/sec; and in the third phase 35 mL saline flush was administered at 4 mL/sec (maximal total volume of contrast medium 110 mL). At the time of the scan, a region of interest was placed in the right ventricular cavity to detect the pre-assigned peak of contrast enhancement, typically 150 Hounsfield Unit (HU). Scans were performed during breath-hold; patients were monitored continuously through single-lead electrocardiography. As clinically indicated, patients were pre-treated with a β-blocker (metoprolol) up to 5 mg intravenously to lower the heart rate to a value below 65 beats per minute (bpm), and with a nitrate (isosorbide dinitrate) up to 1 mg intravenously to determine vasodilatation just prior to CT imaging.
EFV measurement
The images acquired for NCT and coronary CTA were reconstructed with a slice thickness of 2.5 mm and slice distance of 2.5 mm without overlap. DICOM images were transferred to a research workstation for fat quantification. Users were asked to trace the pericardial contours (15 points at least per boundary) in multi-planar reformat (MPR) planes, corresponding to 2- and 4-chamber views, and short axis (SA) views placed at the ventricular base, at the level of the papillary muscles (mid-ventricular region) and at the level of the apex. Boundaries of the computed surface were shown superimposed on the CT images (Figure 1). During the operation, a spline-interpolated curve was shown allowing the user to evaluate the adherence of provided data with the anatomical boundary. In this way, tracing complete curves was avoided which resulted in time saving without significant loss of accuracy. It was possible to draw additional contours in intermediate slices. It was possible to draw additional contours in intermediate slices. In this study, 3 and 6 SA slices were utilized. Furthermore, the procedure allowed the user to split the cardiac region into left and right zones by tracing an inter-ventricular plane in 2 SA views. The superior portion of the pericardial space above the atrioventricular sulcus that included atria and mediastinal vessels was excluded because the computation was very complex and would have required strong manual intervention.
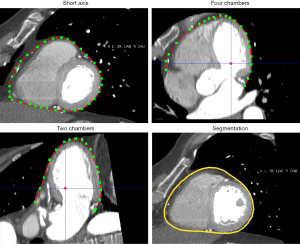
Epicardial surface was computed by using a method previously developed at our Institution (10) that relies on the properties of spherical harmonics (11), which are able to represent any continuous surface of the sphere. Smooth surfaces, such as the epicardial one, can be represented by a reduced number of the terms in the series, resulting in a light computational load. The combination coefficients were estimated by minimizing the distance between the points of the traced contours and the surface itself.
Local manual editing/refinement and re-drawing the boundaries were possible however it was required only in a few cases. EFV was computed from the segmented regions by counting the voxels with a gray level in the range of (−30, −190) HU. This has been validated by previous investigators (12). Results were provided in cubic centimeters (cc). The average gray level and the related standard deviation were also computed.
Experiments were focused to assess the effect of different numbers of SA slices along with the observer variability. Two trained technologists (O1 and O2) having >5 years of experience in cardiac CT and coronary CTA procedures analyzed the images of all patients twice with a time interval between the measurements of at least 2 weeks and with a random order in the presentation of images, with a total of 120 examinations for each operator. The observers were pre-trained to the use of program. In addition, in order to ensure a uniform consensus-based operation, during the training phase, the observers were asked to analyze the traced boundary and the resulting surface.
Statistical analysis
Statistical analyses were performed using Statplus® software. Observer variability was analyzed by Bland-Altman (BA) method (13). Intra-class correlation (ICC) was also evaluated. Statistical correlations were analyzed by Pearson correlation coefficient.
All continuous variables were expressed as median ± inter-quartile range. Differences between samples were tested by the Wilcoxon test. A P value <0.05 was considered statistically significant.
Results
To test the performance of the EFV software, we used CT images from a set of 30 patients referred to our institution for suspected CAD. For each scan, post-processing time took around 2–3 minutes and average computation time was about 10 seconds. The total EFV was 103.62±50.97 and 94.96±67.91 cc in NCT and coronary CTA images respectively, with non-significant difference (P=0.292). Non-significant difference was also observed between EFV computed using 3 and 6 SA slices. A close correlation between EFV measured in NCT and coronary CTA images was found in both operators. EFV measured in coronary CTA was slightly lower than in NCT, the behavior was virtually the same using 3 and 6 SA slices (Figure 2).
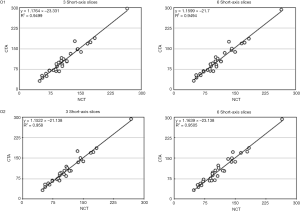
According to Bland and Altman (13), we quantified the intra-observer variability through the Coefficient of Repeatability (CR), which is defined as 1.96 times the standard deviation of the differences between 2 reiterations of measurement. Therefore, difference between 2 observations lies within the range of (−CR, CR) with a probability of 95%. The lower values indicated better repeatability.
When using 3 SA slices, CR error was 10.22 cc for operator-1 and 11.31 cc for operator-2 in NCT, and 7.99 cc for operator-1 and 7.75 cc for operator-2 in coronary CTA (Figure 3). In case of 6 SA slices, CR was 6.33 cc for operator-1 and 6.93 cc for operator-2 in NCT, whereas was 6.07 cc for operator-1 and 6.14 cc for operator-2 in coronary CTA scan (Figure 4).
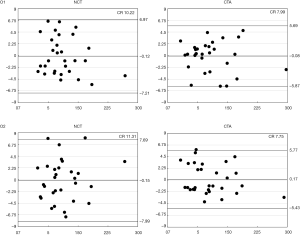
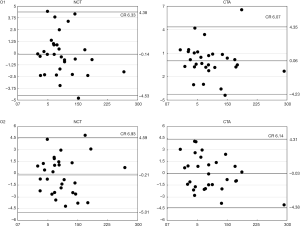
To analyze the inter-observer variability CR and ICC were computed. CR was 8.17 and 8.39 cc in NCT and 7.07 and 7.21 cc in CTA for 6 and 3 SA slices respectively (Table 2). ICC values >0.99 were obtained in all cases (Table 3).
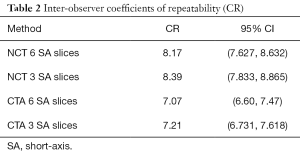
Full table
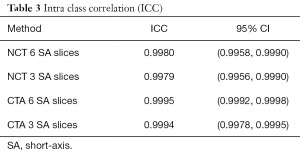
Full table
The right ventricular EFV was 67.23±31.4 and 57.41±34.3 cc, in NCT and coronary CTA respectively; the corresponding values for left ventricular EFV were 38.01±19.1 and 35.27±25.9 cc. The average fat density was −86.6±6.8 HU in NCT vs. −85.4±6.2 HU in CTA, (P<0.04). The range of fat density was (−99.56, −74.1) HU in NCT vs. (−94, −75.82) HU in coronary CTA.
Furthermore, EFV showed a very close correlation with BMI (rho =0.783, P<0.0001), more influenced by the body weight than by the height (Table 4).
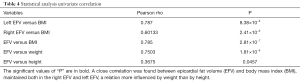
Full table
Discussion
A positive correlation between EFV and coronary plaque burden has been reported in the literature. Also the negative consequences of increased fat deposits in the extra- or intra-pericardial regions and inside the heart (intra- and inter-myocyte cells) on the coronary circulation and on the cardiac function have been reported by previous researchers (14-16). Epicardial and mediastinal adipose tissue can mechanically and functionally compromise the systolic and diastolic function of the cardiac ventricles (17). These evidences explain the importance of a quantitative measurement of the fat volume and the efforts in obtaining tools for diagnostic interpretation and monitoring the relationship between visceral fat and atherosclerotic process.
In this paper, we used a method for semi-automatic EFV measurements, aiming to improve the accuracy of the non-standardized methods generally reported in literature. The latter were limited to manually outlined regions of interest (ROI) and preset density thresholds range for fat tissue, which was subject to inter- and intra-observer and inter-scan variability (6).
The total EFV was 64.38±46.04 and 103.62±50.97 cc (P<10−6) in coronary CTA and NCT, respectively. Those results are similar to those published in the literature, which range from 68±34 to 124±50 mL in population-based studies (18,19) and 110±41 mL in women and 137±53 mL in men in a study including patients from the Framingham cohorts (20).
The average computation time of EFV with our software was about 10-15 seconds after tracing pericardial boundaries, and the overall time needed to complete the measurement was about 2 minutes, while others described procedures that required more time and were thus considered to be excessively time and labor intensive (15,21,22).
To our knowledge, a comparison between measurements of EFV and epicardial fat density (EFD) in cardiac CT with and without CM was not done previously. The results of our study showed a low intra- and inter-observer variability of computer-assisted measurements of EFV in both NCT and coronary CTA, which was similarly found in previous studies (ICC of 0.95) (23,24).
Moreover intra- and inter-observer variability of the computer-assisted measurement of EFV slightly improved with CM. This may be explained by the fact that the contrasted cavities and enhanced vessels allowed a better visualization of the pericardial boundaries (Figure 5). Moreover, in NCT, there may be difficulties to identify the pericardium in lean individuals with less pericardial fat (19).
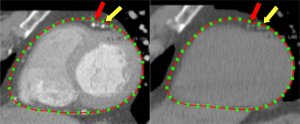
In addition, by increasing the number of SA slices from 3 to 6, it was possible to slightly decrease observer variability.
The average fat density was slightly lower in the series without CM than with CM. Furthermore, fat density range was larger in the series without CM than with CM. Partial volume effect may account for the slight observed differences between measurement done in scan with and without CM. It occurs whenever a structure is only partly within the imaging section (voxel), thereby the densities of the structure and the adjacent or surrounding structures are averaged.
Furthermore, the software for epicardial fat analysis permits a systematic evaluation of total epicardial fat burden, and the segmentation between right and left ventricle. As expected, the EFV values corresponding to the right ventricle were larger than the left one, which is as expected according to the anatomical features.
The analysis of the data showed a close correlation between EFV and BMI, a relationship more influenced by weight than by height, linking EFV with other visceral fat depots. Moreover, the association between EFV and BMI was maintained in both the right EFV and left EFV, which indicate a non-selective accumulation of epicardial fat between the ventricles with the increase in body weight.
The limitation of the study is that population was small (30 patients), with a majority of males (73%) generally overweight (BMI 25.9±6.3).
Conclusions
CT may allow a fast and reliable quantification of EFV in clinical setting, a potential additional predictor of prognosis in patients with CVD. Both NCT and coronary CTA can be used to measure EFV with low intra- and inter-observer variability.
Acknowledgements
None.
Footnote
Conflicts of Interest: The authors have no conflicts of interest to declare.
Disclaimer: The method for determining the volume of epicardial fat from CT images used in this study was patented by European Patent Office (EP2618307) in 2013.
References
- Otaki Y, Rajani R, Cheng VY, Gransar H, Nakanishi R, Shmilovich H, Nakazato R, Hayes SW, Thomson LE, Friedman JD, Slomka PJ, Wong ND, Rozanski A, Shaw L, Budoff M, Berman DS, Dey D. The relationship between epicardial fat volume and incident coronary artery calcium. J Cardiovasc Comput Tomogr 2011;5:310-6. [Crossref] [PubMed]
- Taguchi R, Takasu J, Itani Y, Yamamoto R, Yokoyama K, Watanabe S, Masuda Y. Pericardial fat accumulation in men as a risk factor for coronary artery disease. Atherosclerosis 2001;157:203-9. [Crossref] [PubMed]
- Baker AR, Silva NF, Quinn DW. Human epicardial adipose tissue expresses a pathogenic profile of adipocytokines in patients with cardiovascular disease. Cardiovasc Diabetol 2006;5:1. [Crossref] [PubMed]
- Jeong JW, Jeong MH, Yun KH, Oh SK, Park EM, Kim YK, Rhee SJ, Lee EM, Lee J, Yoo NJ, Kim NH, Park JC. Echocardiographic epicardial fat thickness and coronary artery disease. Circ J 2007;71:536-9. [Crossref] [PubMed]
- Natale F, Tedesco MA, Mocerino R, de Simone V, Di Marco GM, Aronne L, Credendino M, Siniscalchi C, Calabrò P, Cotrufo M, Calabrò R. Visceral adiposity and arterial stiffness: echocardiographic epicardial fat thickness reflects, better than waist circumference, carotid arterial stiffness in a large population of hypertensives. Eur J Echocardiogr 2009;10:549-55. [Crossref] [PubMed]
- Yong HS, Kim EJ, Seo HS, Kang EY, Kim YK, Woo OH, Han H. Pericardial fat is more abundant in patients with coronary atherosclerosis and even in the non-obese patients: evaluation with cardiac CT angiography. Int J Cardiovasc Imaging 2010;26 Suppl 1:53-62. [Crossref] [PubMed]
- Iacobellis G, Ribaudo MC, Assael F, Vecci E, Tiberti C, Zappaterreno A, Di Mario U, Leonetti F. Echocardiographic epicardial adipose tissue is related to anthropometric and clinical parameters of metabolic syndrome: a new indicator of cardiovascular risk. J Clin Endocrinol Metab 2003;88:5163-8. [Crossref] [PubMed]
- Nakazato R, Shmilovich H, Tamarappoo BK, Cheng VY, Slomka PJ, Berman DS, Dey D. Interscan reproducibility of computer-aided epicardial and thoracic fat measurement from noncontrast cardiac CT. J Cardiovasc Comput Tomogr 2011;5:172-9. [Crossref] [PubMed]
- Schlett CL, Hoffmann U. Identification and quantification of fat compartments with CT and MRI and their importance. Radiologe 2011;51:372-8. [Crossref] [PubMed]
- Coppini G, Favilla R, Moroni D, Salvetti O, D’Errico L, Salituri F, Ciardetti M, Schlueter M, Faggioni L, Coceani M, Mazzarisi A, Bianchi M, Bartolozzi C, Marraccini P. Epicardial fat volume assessment in cardiac CT. Int J CARS 2012;7:S40.
- Mousa M, Chaine R, Akkouche S, Galin E. Efficient Spherical Harmonics Representation of 3D Objects. The Visual Computer 2007;23:248-57. (Proceedings of Pacific Graphics).
- Yoshizumi T, Nakamura T, Yamane M, Hasan A, Waliul Islam M, Menju M, Yamasaki K, Arai T, Kotani K, Funahashi T, Yamashita S, Matsuzawa Y. Abdominal Fat: Standardized Technique for Measurement at CT. Radiology 1999;211:283-6. [Crossref] [PubMed]
- Bland JM, Altman DG. Statistical methods for assessing agreement between two methods of clinical measurement. Lancet 1986;1:307-10. [Crossref] [PubMed]
- Ding J, Kritchevsky SB, Harris TB, Burke GL, Detrano RC, Szklo M, Jeffrey Carr J. Multi-Ethnic Study of Atherosclerosis. The association of pericardial fat with calcified coronary plaque. Obesity (Silver Spring) 2008;16:1914-9. [Crossref] [PubMed]
- Alexopoulos N, Dalton S. McLeana, Matthew Janika, Chesnal D. Arepallib, Arthur E. Stillmanb, Paolo Raggia. Epicardial adipose tissue and coronary artery plaque characteristics. Atherosclerosis 2010;210:150-4. [Crossref] [PubMed]
- Rajani R, Shmilovich H, Nakazato R, Nakanishi R, Otaki Y, Cheng VY, Hayes SW, Thomson LE, Friedman JD, Slomka PJ, Min JK, Berman DS, Dey D. Relationship of epicardial fat volume to coronary plaque, severe coronary stenosis, and high-risk coronary plaque features assessed by coronary CT angiography. J Cardiovasc Comput Tomogr 2013;7:125-32. [Crossref] [PubMed]
- Khawaja T, Greer C, Chokshi A, Chavarria N, Thadani S, Jones M, Schaefle K, Bhatia K, Collado JE, Shimbo D, Einstein AJ, Schulze PC. Epicardial fat volume in patients with left ventricular systolic dysfunction. Am J Cardiol 2011;108:397-401. [Crossref] [PubMed]
- Mahabadi AA, Massaro JM, Rosito GA, Levy D, Murabito JM, Wolf PA, O'Donnell CJ, Fox CS, Hoffmann U. Association of pericardial fat, intrathoracic fat, and visceral abdominal fat with cardiovascular disease burden: the Framingham Heart Study. Eur Heart J 2009;30:850-6. [Crossref] [PubMed]
- Ding J, Kritchevsky SB, Hsu FC, Harris TB, Burke GL, Detrano RC, Szklo M, Criqui MH, Allison M, Ouyang P, Brown ER, Carr JJ. Association between non-subcutaneous adiposity and calcified coronary plaque: a sub-study of the Multi-Ethnic Study of Atherosclerosis. Am J Clin Nutr 2008;88:645-50. [PubMed]
- Rosito GA, Massaro JM, Hoffmann U, Ruberg FL, Mahabadi AA, Vasan RS, O'Donnell CJ, Fox CS. Pericardial fat, visceral abdominal fat, cardiovascular disease risk factors,and vascular calcification in a community-based sample: the Framingham Heart Study. Circulation 2008;117:605-13. [Crossref] [PubMed]
- Abbara S, Desai JC, Cury RC, Butler J, Nieman K, Reddy V. Mapping epicardial fat with multi-detector computed tomography to facilitate percutaneous transepicardial arrhythmia ablation. Eur J Radiol 2006;57:417-22. [Crossref] [PubMed]
- Nichols JH, Samy B, Nasir K, Fox CS, Schulze PC, Bamberg F, Hoffmann U. Volumetric measurement of pericardial adipose tissue from contrast-enhanced coronary computed tomography angiography: a reproducibility study. J Cardiovasc Comput Tomogr 2008;2:288-95. [Crossref] [PubMed]
- Fox CS, Gona P, Hoffmann U, Porter SA, Salton CJ, Massaro JM, Levy D, Larson MG, D'Agostino RB Sr, O'Donnell CJ, Manning WJ. Pericardial fat, intrathoracic fat, and measures of left ventricular structure and function: the Framingham Heart Study. Circulation 2009;119:1586-91. [Crossref] [PubMed]
- Gorter PM, de Vos AM, van der Graaf Y, Stella PR, Doevendans PA, Meijs MF, Prokop M, Visseren FL. Relation of epicardial and pericoronary fat to coronary atherosclerosis and coronary artery calcium in patients undergoing coronary angiography. Am J Cardiol 2008;102:380-5. [Crossref] [PubMed]