Diabetes or calcium channel blocker contribute to cerebral hemodynamics after bypass surgery in adult patients with moyamoya disease
Introduction
Moyamoya disease (MMD) is a chronic cerebrovascular disease characterized by terminal stenosis or occlusion of the bilateral internal carotid artery and the formation of an abnormal vascular network at the base of the brain (1). Japan, Korea, and China have had the highest prevalence and incidence of MMD in recent years (2,3). Notably, disease progression is associated with the development of ischemic or hemorrhagic stroke, which are leading causes of death and severe disability (2). Ischemic MMD occurs mostly in children, while hemorrhagic MMD occurs more often in adults, which affected approximately 20% of adult MMD patients (3,4). Thus far, no specific treatment therapy for the prevention or reversal of MMD has been developed, although surgery is widely used to primarily improve cerebral blood flow with hypoperfusion (5). Despite the availability of several surgical methods, the most frequently used methods are inseparable from intracranial and extracranial vessel bypass, such as superficial temporal artery to middle cerebral artery (STA-MCA) bypass, encephalo-duro-myo-arterio-pericranial-synangiosis, encephalo-duro-myo-arterio-synangiosis, encephalo-duro-arterio-synangiosis (EDAS), encephalo-arterio-synangiosis, and encephalo-myo- synangiosis, among others (3,6).
Various types of perfusion imaging have become critical for evaluating cerebral hemodynamic changes and the reserve of the cerebrovascular system prior to and following revascularization. Particularly, perfusion imaging can be used to evaluate the therapeutic effect of revascularization based on quantitative indexes of cerebral hemodynamics (4). Computed tomography perfusion (CTP) is a rapid and noninvasive imaging technique that enables the quantification of perfusion parameters (4). However, to date, only a handful of studies have described the risk factors of hemodynamics (7,8). Therefore, the present study aimed to investigate the effect of different factors on cerebral hemodynamics after bypass surgery in patients with MMD. We present the following article in accordance with the STROBE reporting checklist (available at https://qims.amegroups.com/article/view/10.21037/qims-22-407/rc).
Methods
Patient recruitment
This study protocol was reviewed and approved by the Clinical Research Ethics Committee of Renmin Hospital of Wuhan University (No. WDRY2021-K070). An informed consent exemption was obtained for this study by the Clinical Research Ethics Committee of our hospital. And the study was conducted in accordance with the Declaration of Helsinki (as revised in 2013). This is a retrospective cohort study. We retrieved information for 365 patients who were diagnosed with MMD via computed tomography angiography (CTA) or digital subtraction angiography (DSA) in the Department of Neurosurgery, Renmin Hospital of Wuhan University between January 2015 and June 2021 (Figure 1). We followed up with all enrolled patients by phone in January 2022. Patients were included if they (I) were diagnosed with MMD by CTA/DSA, (II) were aged ≥18 years, and (III) received treatment and follow-up at Renmin Hospital of Wuhan University. Patients were excluded if (I) they had other serious cardiovascular and cerebrovascular diseases and/or (II) if key clinical data were missing. The cohort’s ages ranged from 19 to 68 years. After excluding patients who underwent conservative or nonrevascularization treatment, we collected relevant clinical data for 233 cases of MMD who accepted cerebral revascularization. Both clinical and perfusion imaging data for these patients were retrospectively analyzed, and the outcomes of 217 patients were followed up via phone or at the clinic. The remaining 16 patients were lost to follow-up, which meant that these patients were excluded in the analysis of follow-up data. Diagnostic criteria were developed based on the Japanese Research Committee’s 2012 Pathology and Treatment of Spontaneous Occlusion of the Circle of Willis guidelines for the diagnosis and treatment of MMD (9). For the same patient, the ratio of cerebral perfusion on the operative side to the contralateral side can accurately reflect changes in cerebral hemodynamics after surgery. Therefore, we did not individually analyze the hemispheres but rather compared the CTP values between the hemispheres of the same person. However, the limitation of this analytical method is that the cerebral hemodynamics of the nonsurgical side may also change after revascularization, but the amount of change should be small. In all 217 patients, CTP was reviewed within 1 year following the bypass.
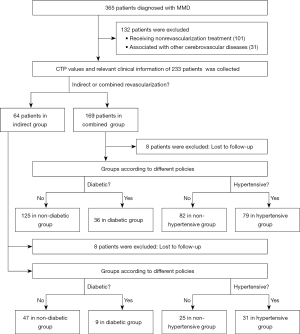
Revascularization modalities
Each patient only received 1 bypass operation. Revascularization procedures included indirect bypass or indirect combined with direct bypass. There were several criteria for surgery, such as transient ischemic attacks and infarcts, severe stenosis of the internal carotid artery or its branches, and an impaired cerebrovascular reserve or cerebral hemorrhage. Neurosurgeons make personalized treatment plans according to the patient’s condition. We evaluated the condition of patients before and during the surgery. Combined revascularization was the first choice when patients had suitable donor or recipient arteries. We confirmed the patency of anastomosed arteries 1–3 days after revascularization by CTA.
Patient groups
Patients with MMD who underwent both STA-MCA and indirect bypass were included in the combined group. And patients who received only indirect bypass were included in the indirect group. A separate method of grouping was adopted to include all patients with MMD and diabetes who had undergone arbitrary bypass surgery in the diabetic group. These were also stratified into either hypertensive or nonhypertensive groups depending on whether or not they had hypertension.
Outcome measures
The examined outcome measures included postoperative complications and neurological status at discharge and at the latest follow-up. All patients who showed neurological changes underwent additional radiographic appraisal by means of computed tomography (CT) or magnetic resonance imaging (MRI) to preclude postoperative ischemic or hemorrhagic complications. Patients’ data, including their gender, age, and risk factors, among others, were retrospectively collected from the medical records and neurosurgical database. Cerebral hyperperfusion syndrome (CHS) was characterized by extreme migraines, seizures, and new neurological deficiencies after the operation. CHS was also characterized by the absence of clear hematomas and the absence of distinct, intense areas of dead tissue on the cerebral MRI or/and CT images (10). We identified diabetes mellitus based on the demonstrative models from the Chinese Rules for the Counteraction and Treatment of Type 2 Diabetes Mellitus (11). A patient was considered to have diabetes mellitus if they had a period discovery of plasma glucose level ≥11.1 mmol·L−1, fasting blood glucose (FBG) ≥7.0 mmol·L−1, or oral glucose resistance test (OGTT) 2 h glucose ≥11.1 mmol·L−1. Hemoglobin A1c (HbA1c) was used to stage diabetes (12). For stage 1, HbA1c was 5.7–6.5%. For stage 2, HbA1c was 6.5–9.0%. For stage 3, HbA1c was above 9.0%. For stage 4, HbA1c was 12% and/or the patient had a diabetic emergency. In our study, we grouped stages 1 and 2 into a new category called Stage I, while we groups stages 3 and 4 into a new category called State II. Good glucose control during the perioperative period meant a fasting plasma glucose level of less than 140 mg/dL (7.8 mmol/L) (13). We diagnosed hypertension using the diagnostic criteria from the 2020 International Society of Hypertension (ISH) Global Hypertension Practice Guidelines (14). The ISH designated an office blood pressure reading of more than 140/90 mmHg as hypertensive. All patients were followed up at the clinic for a modified Rankin Scale (mRS) score, with a mean follow-up time of 48.3±12.5 months. In our study, we grouped mRS 0, 1 and 2 into a new category called mRS I, while we groups mRS 3, 4 and 5 into a new category called mRS II.
Imaging protocols and CTP values
Patients underwent CTP 1 month prior to surgery and were reviewed within 1 year after surgery. Assessment of cerebral perfusion was performed by 2 investigators. CTP values were investigated independently at a workstation using a CT program (GE CT perfusion V. 4.4.2; program: AW Volume Offer 2); following this, the blood volume (CBV), cerebral blood stream (CBF), time to peak (TTP), and mean travel time (MTT) were determined. Two experienced investigators manually delineated regions of interest (ROIs). ROIs were defined in the brain tissue adjacent to the hemorrhagic foci for patients who exhibited simple intraventricular hemorrhage. We set the same pre- and postoperative ROIs by controlling the length and width of the ROIs, the distance from the midline to the ROIs, and the distance from the front of the frontal lobe to the ROIs. Notably, bleeding, calcification, and large vessel passages were not involved in the ROIs. Contralateral mirror ROIs were obtained using a mirror surface. Representative imaging data from 2 typical patients are shown in Figures 2,3. CTP values included CBF, CBV, MTT, and TTP. Moreover, we also measured differences in brain perfusion values before and after surgery. We defined the relative CTP values (rCTP) as the ratio of CTP values on the surgical side to those on the other side, while δCTP values were defined as the ratio of postoperative rCTP values to preoperative rCTP values. The respective formulae were as follows: rCTP=surgical side CTP value/contralateral mirroring areas CTP value, δCTP=post-rCTP/pre-rCTP.
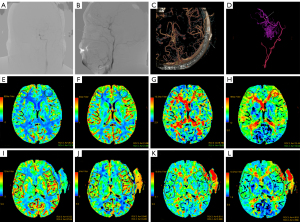
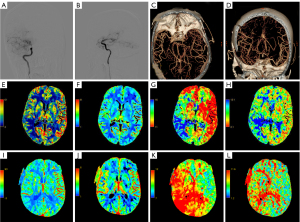
Statistical analysis
All statistical analyses were performed using SPSS for Windows, version 23.0 (IBM Corp.). According to the results of the previous research group pretest, we assumed respectively that the average δMTT detected by the joint group and the indirect group could reach 0.6 and 0.7. The standard deviation was set to 0.2, the β equaled 0.2, the grasp (power = 1−β) equaled 80%, and the bilateral α of the significance level was 0.05. These values were calculated according to the shedding rate of 20%, using PASS 11.0 software (NCSS LLC). Finally, 78 cases were included in each group, with a total of 156 cases in the combined and indirect groups. Data were first tested for normality using the 1-sample Kolmogorov-Smirnov test, and those conforming to a normal distribution are expressed as the mean ± standard deviation. On the other hand, data that were not normally distributed are expressed as median (interquartile range). Differences between 2 groups were analyzed using the chi-square test or the continuity correction of Pearson chi-square tests for categorical data. Otherwise, the Fisher exact test was applied. Continuous variables were analyzed via a t test. Differences in pre- and postoperative CTP values between 2 groups were assessed using a paired t test (normal distribution) or a nonparametric t test (skewed distribution). Data with P values less than 0.05 were considered statistically significant.
Results
Baseline characteristics
We found no statistically significant differences in the baseline characteristics of age, gender, types of stroke, early symptoms, Suzuki stage, history of hypertension, history of diabetes mellitus, history of hyperlipidemia, or hospitalization times between patients in the indirect and combined groups (Table 1). Notably, 64 out of the 233 patients (27.5%) received indirect revascularization. The mean ages of patients in the indirect and combined groups were 46.06±10.19 and 47.23±11.08 years, respectively, and these were not significantly different (P=0.464).
Table 1
Characteristics | Indirect group, n=64 | Combined group, n=169 | P value |
---|---|---|---|
Age (years) | 46.06±10.19 | 47.23±11.08 | 0.464 |
Gender (female) | 36 (56.3%) | 97 (57.4%) | 0.875 |
Type of stroke | – | – | 0.907 |
Ischemic | 48 (75%) | 128 (75.7%) | – |
Hemorrhagic | 16 (25%) | 41 (24.3%) | – |
Early symptoms | – | – | 0.850 |
Giddiness/headache/numbness of limb | 36 (56.3%) | 90 (53.3%) | – |
Focal neurological deficits* | 24 (37.5%) | 70 (41.4%) | – |
Decreased level of consciousness | 4 (6.2%) | 9 (5.3%) | – |
Suzuki stage | – | – | 0.479 |
Suzuki 1 | 2 (3.1%) | 5 (2.9%) | – |
Suzuki 2 | 4 (6.3%) | 9 (5.3%) | – |
Suzuki 3 | 21 (32.8%) | 66 (39.1%) | – |
Suzuki 4 | 21 (32.8%) | 63 (37.3%) | – |
Suzuki 5 | 11 (17.2%) | 13 (7.7%) | – |
Suzuki 6 | 5 (7.8%) | 13 (7.7%) | – |
History of hypertension | 33 (51.6%) | 83 (49.1%) | 0.738 |
History of diabetes mellitus | 12 (18.8%) | 35 (20.7%) | 0.739 |
History of hyperlipidemia | 8 (12.5%) | 27 (16.0%) | 0.507 |
Hospitalization time (days) | 28.63±8.30 | 28.98±9.28 | 0.787 |
Data are presented as mean ± standard deviation or n (%). *, focal neurological deficits, including muscle weakness, aphasia, blurred vision, and memory loss.
Clinical outcomes
Similarly, no statistically significant differences were observed in the clinical outcomes between patients in the indirect and combined groups (Table 2). Notably, none of the 233 patients included in this study had serious postoperative complications. Although some complications resulted from transient hemodynamic changes after revascularization, none of the complications affected long-term cerebral hemodynamics. Moreover, there were no statistically significant differences in the mRS at discharge (P=0.142) or mRS at follow-up (P=0.066) between patients in the indirect and combined groups. Even though there was no statistical significance, we found a higher rate of patients with mRS 3–5 in the indirect group than in the combined group.
Table 2
Clinical outcomes | Indirect group, n=64 | Combined group, n=169 | Statistical data | |
---|---|---|---|---|
χ2 | P value | |||
Improvement of symptoms | 52 (81.3%) | 130 (76.9%) | 0.508 | 0.476 |
mRS at discharge II (n=233) | 16 (25%) | 28 (16.6%) | 2.155 | 0.142 |
mRS at follow-up II (n=217) | 12 (21.4%) | 18 (10.7%) | 3.390 | 0.066 |
Postoperative cerebral infarction | 8 (12.5%) | 15 (8.9%) | 0.685 | 0.408 |
Postoperative epilepsy | 8 (12.5%) | 13 (7.7%) | 1.308 | 0.253 |
Postoperative hyperperfusion syndrome | 4 (6.3%) | 7 (4.1%) | 0.110 | 0.498 |
The mRS II represents scores of 3, 4, and 5. Data are presented as numbers. mRS, modified Rankin Scale.
Comparison in CTP values between the indirect vs. combined groups
Different surgical methods had a similar effect on the improvement of postoperative cerebral hemodynamics in patients with MMD (Table 3). δCBV values were significantly higher in the combined group [1.01 (0.87–1.75)] relative to those in the indirect group [1.34 (1.01–1.63); P=0.027]. Particularly, we found no significant differences in the δCBF (1.67±0.40 vs. 1.91±2.74; P=0.514), δMTT [0.58 (0.43–0.82) vs. 0.68 (0.49–0.84); P=0.291], or δTTP [0.84 (0.78–0.88) vs. 0.79 (0.71–0.99); P=0.538] between the indirect and combined groups.
Table 3
CTP values | Indirect group, n=56 | Combined group, n=161 | P value |
---|---|---|---|
δCBF | 1.67±0.40 | 1.91±2.74 | 0.514 |
δCBV | 1.01 (0.87–1.75) | 1.34 (1.01–1.63) | 0.027 |
δMTT | 0.58 (0.43–0.82) | 0.68 (0.49–0.84) | 0.291 |
δTTP | 0.84 (0.78–0.88) | 0.79 (0.71–0.99) | 0.538 |
Data are presented as mean ± standard deviation or median (interquartile range). δCTP = post-rCTP/pre-rCTP. CTP, computed tomography perfusion; CBF, cerebral blood stream; CBV, cerebral blood volume; MTT, mean travel time; TTP, time to peak.
Comparison between pre-CTP values and post-CTP values in the combined group
We verified that the CTP value could be used as an index to evaluate cerebral hemodynamics after revascularization in patients with MMD in the combined group (Table 4). Results revealed significantly lower rCBF at preoperation [0.57 (0.48–0.70)] relative to postoperation [0.83 (0.71–0.99); P<0.001]. We also found statistically significant differences in preoperative δCBV [0.90 (0.76–1.07) vs. 1.10 (0.96–1.21); P<0.001], δMTT [1.50 (1.31–2.02) vs. 1.03 (0.86–1.22); P<0.001], and rTTP [1.60 (1.38–2.05) vs. 1.32 (1.17–1.48); P<0.001] compared to postoperative CTP values.
Table 4
CTP values | Preoperative CTP values | Postoperative CTP values | P value |
---|---|---|---|
rCBF | 0.57 (0.48–0.70) | 0.83 (0.71–0.99) | <0.001 |
rCBV | 0.90 (0.76–1.07) | 1.10 (0.96–1.21) | <0.001 |
rMTT | 1.50 (1.31–2.02) | 1.03 (0.86–1.22) | <0.001 |
rTTP | 1.60 (1.38–2.05) | 1.32 (1.17–1.48) | <0.001 |
Data are presented as median (interquartile range). CTP, computed tomography perfusion; CBF, cerebral blood stream; CBV, cerebral blood volume; MTT, mean travel time; TTP, time to peak.
Comparison between pre-CTP values and post-CTP values in the indirect group
We verified that the CTP values could be used as an indicator to evaluate cerebral hemodynamics after revascularization in patients with MMD in the indirect group (Table 5). Results revealed significantly lower rCBF preoperation [0.55 (0.45–0.69)] relative to postoperation [0.91 (0.71–1.18); P<0.001]. We also found statistically significant differences in preoperative δCBV [0.92 (0.79–1.26) vs. 1.14 (0.89–1.38); P=0.001], δMTT [1.64 (1.37–2.13) vs. 1.01 (0.77–1.27); P<0.001], and rTTP [1.31 (1.21–1.35) vs. 1.06 (1.01–1.12); P<0.001] compared to postoperative CTP values.
Table 5
CTP values | Preoperative CTP values | Postoperative CTP values | P value |
---|---|---|---|
rCBF | 0.55 (0.45–0.69) | 0.91 (0.71–1.18) | <0.001 |
rCBV | 0.92 (0.79–1.26) | 1.14 (0.89–1.38) | 0.001 |
rMTT | 1.64 (1.37–2.13) | 1.01 (0.77–1.27) | <0.001 |
rTTP | 1.31 (1.21–1.35) | 1.06 (1.01–1.12) | <0.001 |
Data are presented as median (interquartile range). CTP, computed tomography perfusion; CBV, cerebral blood volume; CBF, cerebral blood stream; TTP, time to peak; MTT, mean travel time.
Comparison in δCTP values between the diabetic and nondiabetic groups
To avoid bias caused by the surgical approach, we first analyzed CTP values in the combined group. Cerebral hemodynamics were significantly improved in patients with MMD and diabetes after revascularization relative to those without diabetes (Table 6; Figure 4). Notably, δMTT values were significantly lower in the diabetic group (0.49 (0.35–0.70)) relative to those in the nondiabetic group [0.72 (0.52–0.87); P<0.001]. On the other hand, we found no statistically significant differences in δCBF [1.52 (1.13–2.00) vs. 1.41 (1.17–1.67); P=0.644], δCBV (1.21±0.45 vs. 1.36±0.48; P=0.112), or δTTP [0.77 (0.71–1.01) vs. 0.79 (0.70–0.98); P=0.948] between patients in the diabetic and nondiabetic groups. We also analyzed CTP values from the indirect group and found consistent results (Table 7; Figure 5). We found statistically significant differences in δMTT [0.41 (0.31–0.45) vs. 0.58 (0.48–0.81); P<0.001] between patients in the stage I and stage II groups (Table 8). We also found statistically significant differences in δMTT [0.36 (0.25–0.43) vs. 0.54 (0.47–0.79); P<0.001] between patients in the good glucose control and bad glucose control groups (Table 9).
Table 6
CTP values | Diabetic group, n=36 | Nondiabetic group, n=125 | Statistical data | |
---|---|---|---|---|
t | P value | |||
δCBF | 1.52 (1.13–2.00) | 1.41 (1.17–1.67) | – | 0.644 |
δCBV | 1.21±0.45 | 1.36±0.48 | 1.596 | 0.112 |
δMTT | 0.49 (0.35–0.70) | 0.72 (0.52–0.87) | – | <0.001 |
δTTP | 0.77 (0.71–1.01) | 0.79 (0.70–0.98) | – | 0.948 |
Data are presented as mean ± standard deviation or median (interquartile range). δCTP = post-rCTP/pre-rCTP. CTP, computed tomography perfusion; CBV, cerebral blood volume; CBF, cerebral blood stream; TTP, time to peak; MTT, mean travel time.
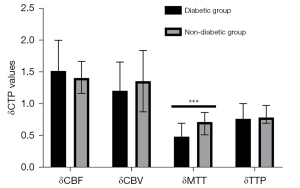
Table 7
CTP values | Diabetic group, n=9 | Nondiabetic group, n=47 | Statistical data | |
---|---|---|---|---|
t | P value | |||
δCBF | 1.90±0.37 | 1.62±0.40 | 1.895 | 0.064 |
δCBV | 1.13 (1.09–1.21) | 0.93 (0.84–1.93) | – | 0.304 |
δMTT | 0.49 (0.35–0.46) | 0.76 (0.44–0.84) | – | 0.007 |
δTTP | 0.84±0.03 | 0.82±0.07 | 1.254 | 0.221 |
Data are presented as mean ± standard deviation or median (interquartile range). δCTP=post-rCTP/pre- rCTP. CTP, computed tomography perfusion; CBV, cerebral blood volume; CBF, cerebral blood stream; TTP, time to peak; MTT, mean travel time.
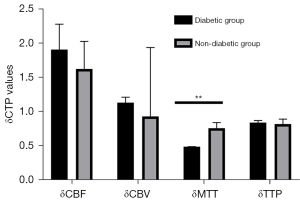
Table 8
CTP values | Stage I group, n=26 | Stage II group, n=19 | Statistical data | |
---|---|---|---|---|
t | P value | |||
δCBF | 1.52 (1.08–2.06) | 1.69 (1.33–2.00) | – | 0.476 |
δCBV | 1.12±0.29 | 1.31±0.51 | 1.583 | 0.121 |
δMTT | 0.41 (0.31–0.45) | 0.58 (0.48–0.81) | – | <0.001 |
δTTP | 0.79 (0.73–0.89) | 0.88 (0.72–1.01) | – | 0.161 |
Data are presented as mean ± standard deviation or median (interquartile range). δCTP=post-rCTP/pre-rCTP. CTP, computed tomography perfusion; CBV, cerebral blood volume; CBF, cerebral blood stream; TTP, time to peak; MTT, mean travel time.
Table 9
CTP values | Good glucose control group, n=17 | Bad glucose control group, n=28 | Statistical data | |
---|---|---|---|---|
t | P value | |||
δCBF | 1.55 (1.17–2.30) | 1.65 (1.24–1.87) | – | 0.640 |
δCBV | 1.17±0.25 | 1.21±0.48 | 0.368 | 0.714 |
δMTT | 0.36 (0.25–0.43) | 0.54 (0.47–0.79) | – | <0.001 |
δTTP | 0.76 (0.72–0.85) | 0.88 (0.73–1.00) | – | 0.087 |
Data are presented as mean ± standard deviation or median (interquartile range). δCTP=post-rCTP/pre-rCTP. CTP, computed tomography perfusion; CBV, cerebral blood volume; CBF, cerebral blood stream; TTP, time to peak; MTT, mean travel time.
Comparison in δCTP values between the hypertensive and nonhypertensive groups
To avoid bias caused by the surgical approach, we first analyzed CTP values in the combined group. Revascularization improved the cerebral hemodynamics of hypertensive MMD patients to a greater degree than it did in those without hypertension (Table 10; Figure 6). Particularly, δCBV values of patients in the hypertensive group (1.41±0.47) were significantly lower than those in the non-hypertensive group (1.25±0.47; P=0.027). However, we found no statistically significant differences in δCBF [1.49 (1.25–1.83) vs. 1.38 (1.12–1.72); P=0.097], δMTT [0.68 (0.44–0.85) vs. 0.69 (0.51–0.83); P=0.555], or δTTP [0.78 (0.69–0.98) vs. 0.80 (0.71–0.99); P=0.374] between patients in the hypertensive and nonhypertensive groups. We also analyzed CTP values from the indirect group and found consistent results (Table 11; Figure 7). We divided patients into 2 groups based on whether they were taking CCB or not. We found statistically significant differences in δCBV [1.46 (1.10–1.83) vs. 1.12 (0.93–1.54); P=0.011] between patients in the calcium channel antagonist and the other groups (Table 12).
Table 10
CTP values | Hypertensive group, n=79 | Nonhypertensive group, n=82 | Statistical data | |
---|---|---|---|---|
t | P value | |||
δCBF | 1.49 (1.25–1.83) | 1.38 (1.12–1.72) | – | 0.097 |
δCBV | 1.41±0.47 | 1.25±0.47 | 2.225 | 0.027 |
δMTT | 0.68 (0.44–0.85) | 0.69 (0.51–0.83) | – | 0.555 |
δTTP | 0.78 (0.69–0.98) | 0.80 (0.71–0.99) | – | 0.374 |
Data are presented as mean ± standard deviation or median (interquartile range). δCTP=post-rCTP/pre-rCTP. CTP, computed tomography perfusion; CBV, cerebral blood volume; CBF, cerebral blood stream; TTP, time to peak; MTT, mean travel time.
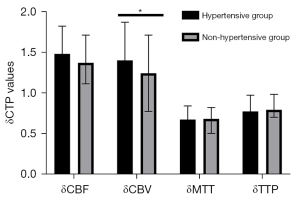
Table 11
CTP values | Hypertensive group, n=31 | Nonhypertensive group, n=25 | Statistical data | |
---|---|---|---|---|
t | P value | |||
δCBF | 1.74±0.33 | 1.58±0.47 | 1.485 | 0.145 |
δCBV | 1.18 (0.93–2.06) | 0.90 (0.84–1.01) | – | 0.002 |
δMTT | 0.48 (0.43–0.82) | 0.76 (0.42–0.81) | – | 0.692 |
δTTP | 0.82±0.07 | 0.84±0.06 | 1.148 | 0.256 |
Data are presented as mean ± standard deviation or median (interquartile range). δCTP=post-rCTP/pre-rCTP. CTP, computed tomography perfusion; CBV, cerebral blood volume; CBF, cerebral blood stream; TTP, time to peak; MTT, mean travel time.
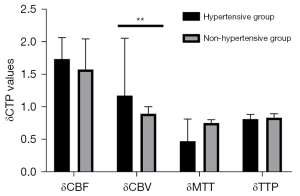
Table 12
CTP values | CCB group, n=74 | Non-CCB group, n=36 | P value |
---|---|---|---|
δCBF | 1.60 (1.35–1.96) | 1.62 (1.21–1.88) | 0.716 |
δCBV | 1.46 (1.10–1.83) | 1.12 (0.93–1.54) | 0.011 |
δMTT | 0.69 (0.44–0.86) | 0.65 (0.43–0.83) | 0.669 |
δTTP | 0.80 (0.71–0.94) | 0.81 (0.71–0.90) | 0.738 |
Data are presented as median (interquartile range). δCTP=post-rCTP/pre-rCTP. CCB, calcium channel blocker; CTP, computed tomography perfusion; CBV, cerebral blood volume; CBF, cerebral blood stream; TTP, time to peak; MTT, mean travel time.
Discussion
Results of the present study revealed that an indirect bypass has similar effects to a combined bypass regarding the postoperative clinical prognosis of patients with MMD. In addition, our findings confirmed that CTP values could be used to evaluate cerebral hemodynamic improvement after revascularization. Interestingly, we found that patients with MMD who underwent revascularization had significantly improved cerebral hemodynamics in the presence of both diabetes and hypertension. These results may be attributed to the patients’ past hyperglycemic status, perioperative hyperglycemic level, or history of hypoglycemic drug use, as well as their prior hypertension, perioperative hypertension level, or history of use of antihypertensive medication. We hypothesized that CCB might promote the improvement of postoperative cerebral hemodynamics in patients with MMD. However, this requires further validation using multicenter cohort studies or randomized controlled clinical trials with larger sample sizes.
It has been established that CHS occurs only after direct revascularization or combined revascularization. However, this study also found 4 cases of CHS after indirect revascularization, which is in line with a few other studies (15,16). Some possible theories for explaining this are as follows. The focal vasodilating effect can be caused by vascular endothelial growth factor and platelet-derived growth factor or neuropeptides (17,18). The subsequent increase in CBF can be a result of focal neuronal excitability, such as insulinlike growth factor 1 and fibroblast growth factor (19,20). However, further research is needed to verify these hypotheses and explore the specific causes.
Revascularization is the most effective treatment therapy for MMD (3). Gradual hemodynamic changes can be observed after indirect revascularization, while spontaneous hemodynamic changes can be obtained after direct bypass surgery. Through a combination of indirect revascularization with direct bypass surgery, vascularization can change either immediately or gradually. When comparing the effect of combined bypass surgery with indirect revascularization, we wanted to evaluate the effect of direct bypass in revascularization. Several studies have suggested that factors such as the patients’ general situation, clinical and imaging characteristics, hemodynamics, metabolic assessment results, and surgical methods that their surgeon is proficient in should be taken into account during surgery (3). Patients with MMD may have a better prognosis after combined bypass surgery (3). Additional evidence suggests that surgeons should protect the spontaneous growth of intracranial and extracranial anastomotic vessels as much as possible because different procedures are equally safe and effective (5). Further, neurosurgeons should use their own experience and strengths to select the most appropriate surgical method for individual treatment. More importantly, they should not only focus on perioperative management but also on the prevention of postoperative complications, which can improve the long-term neurological function of patients (21,22). Further multicenter large-scale prospective randomized controlled clinical trials are needed to verify whether there is a safer and more effective surgical combination or a more updated surgical method for patients with MMD.
The main imaging techniques used to evaluate cerebral hemodynamics include polyethylene terephthalate (PET), single-photon emission computed tomography (SPECT), xenon-enhanced CT, dynamic perfusion CT, magnetic resonance imaging (MRI) with dynamic susceptibility contrast and with arterial spin labeling (ASL), and Doppler ultrasonography (23). SPECT studies have poor spatial resolution, and rCBF values are generated through statistical comparisons from normal controls to represent areas of abnormal perfusion (24). Studies show that PET can be used to assess cerebral oxygen metabolism, rOEF (which represents the fraction of O2 consumed by the brain), and regional CBV (25,26). The main disadvantage of PET imaging is that it is much less available and relatively more costly than other methods (23). Xenon-enhanced CT has been widely used to study MMD for over 30 years (27). The main disadvantages are that it has a high cost, has a relatively long acquisition time, is prone to motion artifacts, and cannot be tolerated by certain patients who cannot endure inhaling xenon during the procedure (23). The ASL MRI modality uses endogenous water as a tracer, so it requires no contrast media (28). Advantages include the ability to repeat the study, the lack of intravenous contrast, the lack of radiation, and the ability to compare CBF values within and between patient examinations. The main disadvantage of using this technique in studying MMD is that the delayed arterial time through the collateral pathway may lead to significant underestimation of CBF and errors due to labeling agent that remains in feeding vessels rather than being extracted into the brain parenchyma (23). Doppler ultrasonography is a noninvasive technique that is very useful and can be performed at the bedside. However, it is very dependent on operators and is prone to inaccurate results (29). The advantages of CTP include the large number of CT scanners installed around the world, the high speed of examination, and the lack of obvious contraindications. Its disadvantages include the fact that radiation doses and iodized contrast agents may not be suitable for some patients. You can easily generate CBF, CBV, and MTT mappings through AW Volume.
CTP, a noninvasive method for examining cerebral blood perfusion, has been shown to effectively evaluate cerebral hemodynamics in patients with cerebrovascular diseases (4,30,31). Notably, age, sex, and clinical types were found to be potential risk factors for cerebral perfusion changes in adult patients with MMD. A previous study demonstrated that chronic CBV of patients with hemorrhagic MMD after revascularization significantly reduced and was relatively stable, while both MTT and TTP were shortened to varying degrees, although there was no significant change in CBF (32). The location and extent of cerebral ischemia can also be determined using CTP, and CTP can better ascertain whether cerebral perfusion is improved after vascular remodeling than other methods (4).
Bower (33) found that diagnosis of the MMD syndrome was markedly delayed because clinicians believed the patients’ symptoms were caused by low blood sugar. For example, in some patients with nonfocal neurological symptoms, MMD syndrome is not diagnosed until the patient develops focal neurological symptoms. Some studies demonstrated that patients with MMD complicated with type 2 diabetes mellitus (T2DM) had a better prognosis and more collateral circulation after EDAS (34). However, the factors influencing collateral vasculogenesis in patients with T2DM complicated with MMD remain unclear, necessitating further research exploration. Results in one study further revealed more frequent collateral formation in the diabetic group, while a wider range of collateral (more than one-third of the middle cerebral artery distribution) was also detected in patients with T2DM. Therefore, the authors speculated that T2DM could promote the formation of lateral branches of pia meningeal vessels in patients with chronic cerebral ischemia, such as MMD (34). This was consistent with the results of our present study. Previous studies also indicate that diabetes-induced neovascularization can generate varying effects in different organ systems. Diabetic retinopathy is characterized by excessive angiogenesis and impaired coronary and peripheral circulation angiogenesis (35-38). These studies suggest that patients with MMD and diabetes have more developed collateral circulation, which may explain the observed reduction in MTT. In the present study, we hypothesized that high perioperative blood glucose levels observed in patients with MMD could improve their hemodynamics by promoting neovascularization.
Although numerous studies have shown that hypertension is a risk factor for both MMD bleeding and postoperative rebleeding, none have examined whether hypertension can improve patient prognosis. To this end, we hypothesized that antihypertensive drugs may play a key role in the influence of postoperative hemodynamic improvement (22). For example, nicardipine was found to have beneficial effects on cerebral hemodynamics, where it could prevent ischemic sequelae by optimizing existing collateral circulation (39). Moreover, the use of verapamil, a calcium channel antagonist, reportedly improved acute neurological dysfunction in patients with MMD (40), while flunarizine was found to improve cerebral perfusion after unilateral MMD (41,42). Taken together, the findings from these studies demonstrate that CCB can improve the prognosis of patients with MDD, which was consistent with our results.
The limitations of this study were that the patient source was a single center, and this study only examined adult patients with MMD. Therefore, prospective clinical studies of large multicenter samples and related studies of childhood MMD are needed in the future. Nonetheless, this single-center study used personalized treatment for patients according to the latest guidelines. Therefore, these results may be used as a reference for other medical centers.
Conclusions
The results of the present study demonstrated that diabetes and CCB effectively improve hemodynamics in patients with MMD after bypass as evidenced by the CTP values. Notably, δMTT values revealed downward trends when patients had diabetes mellitus and δCBV values revealed rising trends when patients have had hypertension while CTP values could be used as a prognostic indicator. Furthermore, it is clear that bypass improved cerebral hemodynamics in patients with MMD, although the effect of different surgical types on prognosis was the same.
Acknowledgments
Funding: This research was funded by the National Natural Science Foundation of China (Nos. 81971870 and 82172173).
Footnote
Reporting Checklist: The authors have completed the STROBE reporting checklist. Available at https://qims.amegroups.com/article/view/10.21037/qims-22-407/rc
Conflicts of Interest: All authors have completed the ICMJE uniform disclosure form (available at https://qims.amegroups.com/article/view/10.21037/qims-22-407/coif). The authors have no conflicts of interest to declare.
Ethical Statement:
Open Access Statement: This is an Open Access article distributed in accordance with the Creative Commons Attribution-NonCommercial-NoDerivs 4.0 International License (CC BY-NC-ND 4.0), which permits the non-commercial replication and distribution of the article with the strict proviso that no changes or edits are made and the original work is properly cited (including links to both the formal publication through the relevant DOI and the license). See: https://creativecommons.org/licenses/by-nc-nd/4.0/.
References
- Phi JH, Wang KC, Lee JY, Kim SK. Moyamoya Syndrome: A Window of Moyamoya Disease. J Korean Neurosurg Soc 2015;57:408-14. [Crossref] [PubMed]
- Kim JS. Moyamoya Disease: Epidemiology, Clinical Features, and Diagnosis. J Stroke 2016;18:2-11. [Crossref] [PubMed]
- Shang S, Zhou D, Ya J, Li S, Yang Q, Ding Y, Ji X, Meng R. Progress in moyamoya disease. Neurosurg Rev 2020;43:371-82. [Crossref] [PubMed]
- Wang X, Chong Z, Guo X, Liu D, Sun Z, Chen Y, Gao L, Chen Y. Evaluation of Hemodynamics Before and After Revascularization in Hemorrhagic Moyamoya Disease: A Computed Tomography Perfusion Imaging Case Study. World Neurosurg 2019;131:e277-83. [Crossref] [PubMed]
- Scott RM, Smith ER. Moyamoya disease and moyamoya syndrome. N Engl J Med 2009;360:1226-37. [Crossref] [PubMed]
- Kalra N, Bautista M, McCullagh H, Tyagi A, Peds QL. Score Post Encephalo-duro-arterio-myo-synangiosis Procedure for Moyamoya Disease: A Single Center Experience. World Neurosurg 2020;144:e674-8. [Crossref] [PubMed]
- Sasagawa A, Mikami T, Hirano T, Akiyama Y, Mikuni N. Characteristics of cerebral hemodynamics assessed by CT perfusion in moyamoya disease. J Clin Neurosci 2018;47:183-9. [Crossref] [PubMed]
- Kuribara T, Mikami T, Komatsu K, Kimura Y, Kim S, Miyata K, Akiyama Y, Enatsu R, Hirano T, Mikuni N. Preoperatively estimated graft flow rate contributes to the improvement of hemodynamics in revascularization for Moyamoya disease. J Stroke Cerebrovasc Dis 2021;30:105450. [Crossref] [PubMed]
- Health Labour Sciences Research Grant for Research on Measures for Infractable Diseases. Guidelines for diagnosis and treatment of moyamoya disease (spontaneous occlusion of the circle of Willis). Neurol Med Chir (Tokyo) 2012;52:245-66. [Crossref] [PubMed]
- Gao F, Zhao W, Zheng Y, Duan Y, Ji M, Lin G, Zhu Z. Intravoxel Incoherent Motion Magnetic Resonance Imaging Used in Preoperative Screening of High-Risk Patients With Moyamoya Disease Who May Develop Postoperative Cerebral Hyperperfusion Syndrome. Front Neurosci 2022;16:826021. [Crossref] [PubMed]
- National Clinical Medical Research Center for Geriatric Diseases (PLA General Hospital). Clinical guidelines for prevention and treatment of type 2 diabetes mellitus in the elderly in China (2022 edition). Zhonghua Nei Ke Za Zhi 2022;61:12-50. [PubMed]
- Mori T, Nagata T, Nagata M, Fujimoto K, Fujino Y, Mori K. Diabetes severity measured by treatment control status and number of anti-diabetic drugs affects presenteeism among workers with type 2 diabetes. BMC Public Health 2021;21:1865. [Crossref] [PubMed]
- Dogra P, Jialal I. Diabetic Perioperative Management. StatPearls. Treasure Island (FL): StatPearls Publishing Copyright © 2022, StatPearls Publishing LLC.; 2022.
- Unger T, Borghi C, Charchar F, Khan NA, Poulter NR, Prabhakaran D, Ramirez A, Schlaich M, Stergiou GS, Tomaszewski M, Wainford RD, Williams B, Schutte AE. 2020 International Society of Hypertension Global Hypertension Practice Guidelines. Hypertension 2020;75:1334-57. [Crossref] [PubMed]
- Cho WS, Lee HY, Kang HS, Kim JE, Bang JS, Oh CW. Symptomatic cerebral hyperperfusion on SPECT after indirect revascularization surgery for Moyamoya disease. Clin Nucl Med 2013;38:44-6. [Crossref] [PubMed]
- Murchison J, Wilson JM, Ray C, Ginsberg J, Nagy L. Moyamoya Disease in an 18-Month-Old Female Caucasian Complicated by Cerebral Hyperperfusion Syndrome Following Indirect Revascularization. Am J Case Rep 2017;18:1077-80. [Crossref] [PubMed]
- Krupinski J, Issa R, Bujny T, Slevin M, Kumar P, Kumar S, Kaluza J. A putative role for platelet-derived growth factor in angiogenesis and neuroprotection after ischemic stroke in humans. Stroke 1997;28:564-73. [Crossref] [PubMed]
- Macfarlane R, Moskowitz MA, Sakas DE, Tasdemiroglu E, Wei EP, Kontos HA. The role of neuroeffector mechanisms in cerebral hyperperfusion syndromes. J Neurosurg 1991;75:845-55. [Crossref] [PubMed]
- Selinfreund RH, Blair LA. Insulin-like growth factor-I induces a rapid increase in calcium currents and spontaneous membrane activity in clonal pituitary cells. Mol Pharmacol 1994;45:1215-20. [PubMed]
- Li AJ, Suzuki S, Suzuki M, Mizukoshi E, Imamura T. Fibroblast growth factor-2 increases functional excitatory synapses on hippocampal neurons. Eur J Neurosci 2002;16:1313-24. [Crossref] [PubMed]
- Ando T, Shimada Y, Fujiwara S, Yoshida K, Kobayashi M, Kubo Y, Terasaki K, Ando S, Ogasawara K. Revascularisation surgery improves cognition in adult patients with moyamoya disease. J Neurol Neurosurg Psychiatry 2020;91:332-4. [Crossref] [PubMed]
- Zhang Q, Zhao M, Ge P, Liu X, Wang R, Zhang Y, Zhang D, Zhao J. Hemorrhagic patterns and their risk factors in patients with moyamoya disease. Eur J Neurol 2020;27:2499-507. [Crossref] [PubMed]
- Lee M, Zaharchuk G, Guzman R, Achrol A, Bell-Stephens T, Steinberg GK. Quantitative hemodynamic studies in moyamoya disease: a review. Neurosurg Focus 2009;26:E5. [Crossref] [PubMed]
- Liming Z, Weiliang S, Jia J, Hao L, Yang L, Ludtka C, Jahromi BR, Goehre F, Zemmar A, Tianxiao L, Hernesniemi J, Andrade-Barazarte H, Chaoyue L. Impact of blood pressure changes in cerebral blood perfusion of patients with ischemic Moyamoya disease evaluated by SPECT. J Cereb Blood Flow Metab 2021;41:1472-80. [Crossref] [PubMed]
- Igarashi C, Okazawa H, Islam MM, Tsujikawa T, Higashino T, Isozaki M, Kikuta KI. Differences in Hemodynamic Alteration between Atherosclerotic Occlusive Lesions and Moyamoya Disease: A Quantitative 15O-PET Study. Diagnostics (Basel) 2021;11:1820. [Crossref] [PubMed]
- Zerweck L, Roder C, Hauser TK, Thurow J, Mengel A, Tatagiba M, Khan N, Meyer PT, Ernemann U, Klose U. Hemodynamic evaluation of patients with Moyamoya Angiopathy: comparison of resting-state fMRI to breath-hold fMRI and 15Owater PET. Neuroradiology 2022;64:553-63. [Crossref] [PubMed]
- Suzuki R, Nariai T, Matsushima Y, Hirakawa K. Xe-CT in cerebrovascular disease and moyamoya disease. Acta Neurol Scand Suppl 1996;166:69-71. [Crossref] [PubMed]
- Kuwabara Y, Ichiya Y, Sasaki M, Yoshida T, Masuda K, Matsushima T, Fukui M. Response to hypercapnia in moyamoya disease. Cerebrovascular response to hypercapnia in pediatric and adult patients with moyamoya disease. Stroke 1997;28:701-7. [Crossref] [PubMed]
- Lee YS, Jung KH, Roh JK. Diagnosis of moyamoya disease with transcranial Doppler sonography: correlation study with magnetic resonance angiography. J Neuroimaging 2004;14:319-23. [Crossref] [PubMed]
- Shi Z, Ma G, Zhang D. Haemodynamic analysis of adult patients with moyamoya disease: CT perfusion and DSA gradings. Stroke Vasc Neurol 2021;6:41-7. [Crossref] [PubMed]
- Dehkharghani S, Andre J. Imaging Approaches to Stroke and Neurovascular Disease. Neurosurgery 2017;80:681-700. [Crossref] [PubMed]
- Kang K, Ma N, Li J, Shen Y, Gu W, Ma G, Zhang D, Zhao X. Cerebral Hemodynamic Changes After Revascularization in Patients With Hemorrhagic Moyamoya Disease. Front Neurol 2020;11:72. [Crossref] [PubMed]
- Bower RS, Mallory GW, Nwojo M, Meyer FB, Kudva YC. Diabetes mellitus and the Moyamoya syndrome. Ann Intern Med 2012;157:387-8. [Crossref] [PubMed]
- Ren B, Zhang ZS, Liu WW, Bao XY, Li DS, Han C, Xian P, Zhao F, Wang H, Wang H, Duan L. Surgical outcomes following encephaloduroarteriosynangiosis in adult moyamoya disease associated with Type 2 diabetes. J Neurosurg 2016;125:308-14. [Crossref] [PubMed]
- Abaci A, Oğuzhan A, Kahraman S, Eryol NK, Unal S, Arinç H, Ergin A. Effect of diabetes mellitus on formation of coronary collateral vessels. Circulation 1999;99:2239-42. [Crossref] [PubMed]
- Hammes HP, Feng Y, Pfister F, Brownlee M. Diabetic retinopathy: targeting vasoregression. Diabetes 2011;60:9-16. [Crossref] [PubMed]
- Lizotte F, Paré M, Denhez B, Leitges M, Guay A, Geraldes P. PKCδ impaired vessel formation and angiogenic factor expression in diabetic ischemic limbs. Diabetes 2013;62:2948-57. [Crossref] [PubMed]
- Costa PZ, Soares R. Neovascularization in diabetes and its complications. Unraveling the angiogenic paradox. Life Sci 2013;92:1037-45. [Crossref] [PubMed]
- Hosain SA, Hughes JT, Forem SL, Wisoff J, Fish I. Use of a calcium channel blocker (nicardipine HCl) in the treatment of childhood moyamoya disease. J Child Neurol 1994;9:378-80. [Crossref] [PubMed]
- McLean MJ, Gebarski SS, van der Spek AF, Goldstein GW. Response of moyamoya disease to verapamil. Lancet 1985;1:163-4. [Crossref] [PubMed]
- Kuroki M, Nagamachi S, Hoshi H, Flores LG 2nd, Ohnishi T, Jinnouchi S, Futami S, Watanabe K. Cerebral perfusion imaging evaluates pharmacologic treatment of unilateral moyamoya disease. J Nucl Med 1996;37:84-6. [PubMed]
- Nakano M, Suzuki T, Shimada H, Uemura O, Mizuguchi K, Yokochi K. The efficacy of the flunarizine in the treatment of ischemic attack of moyamoya disease. No To Hattatsu 1988;20:252-4. [PubMed]